What you will learn in this 32-minute webinar:
- >> Bivalent CAR T cells targeting EGFR and IL13Rα2 show preliminary safety and bioactivity in recurrent glioblastoma (rGBM) in a phase 1 clinical trial.
- >> Glioblastoma organoids (GBOs) are a promising platform for in vitro assessment of CAR T cell bioactivity and insights into immunotherapy efficacy.
- >> Axion Biosystems' Maestro ZHT* live-cell analysis platform can be used to evaluate bioactivity of cell therapies against glioblastoma organoids and monolayers, label-free and in real time.
*Axion BioSystems' Maestro ZHT system was provided under a Loan Agreement.
About the presenter:
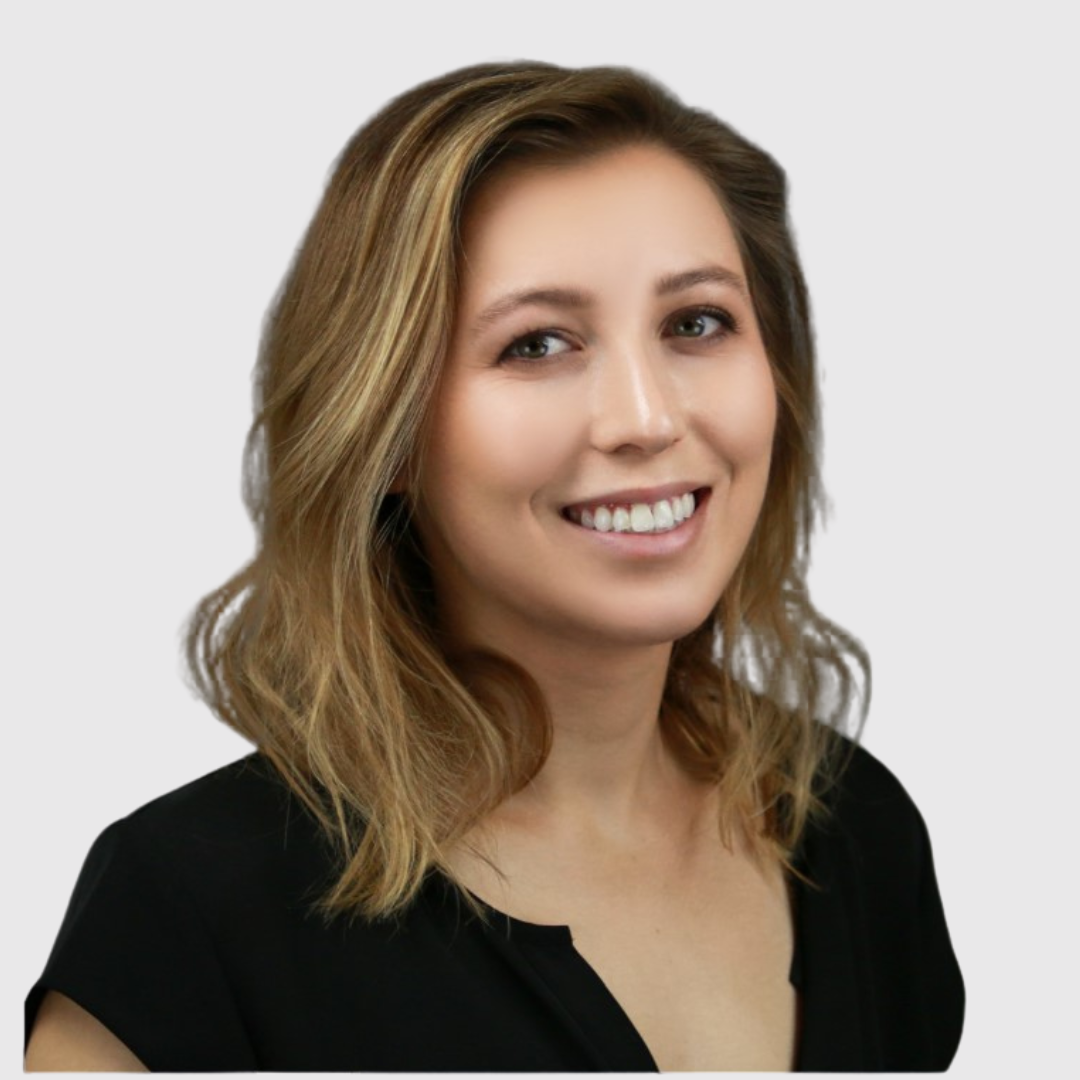
Meghan Logun, PhD
Meghan Logun received her masters of science in Animal Science from Emory University and her PhD in Neuroscience from the University of Georgia under the mentorship of Lohitash Karumbaiah, PhD, exploring the role of extracellular matrix modifications in glioblastoma invasion. She worked as a postdoctoral researcher in Donald O'Rourke's lab at the University of Pennsylvania working on creative delivery approaches for cellular immunotherapies to CNS tumors and has recently transitioned to the NIH/FDA as a postdoc and regulatory oncology product reviewer focusing on cell and gene therapies.
Transcript of the webinar:
Lauren Coyle:
BioInsight's webinar, titled Assessing CAR T-Cell Therapy in Vitro Glioblastoma, was organized by BioInsights. I'm Lauren Coyle, an editor at BioInsights, and joining me today is Meghan Logun, who will discuss promising dual-targeting CAR T-cells for recurrent glioblastoma. She will also share insights on using patient-derived organoids to model treatment response and correlate with clinical outcomes.
After the presentation, we'll have a live Q&A session. We invite our audience to pose their questions to our speaker using the "Ask a Question" box at the bottom of the screen, and we'll try to address them during the session.
Now, I'd like to introduce our presenter. Meghan Logun holds advanced degrees in Animal Science and Neuroscience, with extensive research experience in glioblastoma and cellular immunotherapies. She currently serves as a postdoctoral and regulatory oncology product reviewer at the NIH-FDA, specializing in cell and gene therapies.
Without further ado, I'll hand it over to Meghan to begin her presentation.
Meghan Logun:
Thank you so much for that introduction. Today, I’m going to be talking about one of my postdoctoral projects that I participated in at the University of Pennsylvania. In tandem with our ongoing clinical trial for a bicistronic CAR T-cell, I was able to use patient-derived glioblastoma organoids to study CAR T-cell therapy in vitro.
So for those of you familiar with glioblastoma, you know how devastating this disease can be and how it remains a massive unmet medical need. It is the most common primary malignant brain tumor in adults. Patients have almost a 100% chance of recurrence, and even with all standard-of-care therapies, the average patient survives less than one year.
You might have seen a lot of recent press about advances in cellular immunotherapy, particularly with chimeric antigen receptor (CAR) T-cell therapy, whereby they take the patient’s own T-cells and transduce them to express a T-cell receptor that then targets a tumor-specific or tumor-associated antigen. Even though these have shown really great success in hematologic malignancies, they have not achieved the same success in solid tumors, especially glioblastoma--with one of the biggest issues being that glioblastoma (GBM) is prone to antigen escape and is a highly heterogeneous tumor. So we decided, as a lab who targets glioblastoma, to make a bivalent CAR T-cell. That means that our CAR T-cells have two different chimeric antigen receptors on their surface: one targeting an EGFR variant III tumor-specific mutation as well as the commonly overexpressed IL13 receptor alpha-2.
Separately, these two antigens are prevalent across 50-60% of primary GBM's. This is the product that we are currently testing in a first-in-human Phase 1 trial for recurrent GBM.
We have taken these bivalent CAR T-cells and we have patients who have already received diagnoses of recurrent GBM. They receive a surgical resection here at Penn, and then post-resection they receive CAR T-cell treatment in one of the three different dose escalation in one of the three doses. Then they are followed for long-term survival and bioactivity of these CAR T-cells.
One of the things that interested me as a researcher is that we have these patients coming in receiving surgeries at Penn, and then that tissue is being used to turn into patient-derived organoids. Can we use those in combination with other resources generated from the trial to see if we can create any biomarkers or help with interpretation of how these patients are responding, in real-time, to that treatment?
One of our collaborators, the Song Lab at the University of Pennsylvania, worked with us previously to publish a paper on what we call our patient-derived glioblastoma organoids (or, as I'll call them GBOs moving forward). This pipeline by which we're able to take resected tumor tissue from GBM patients and culture them long-term as these multicellular organoids that retain a lot of the original tumor architecture.
The GBOs are obtained fresh, microdissected, and then we are able to culture them out. We have data up to 12 weeks, where they're cultured before or being stored and biobanked for long-term storage for potential future studies. One of the really cool things about these GBOs is that, in addition to maintaining a lot of the cellular heterogeneity of the parent GBM, they also retain some of the tumor-associated vasculature and hypoxic zones that are hallmark to GBM--which is something that's very hard to re-establish from in vitro cell cultures.
Ultimately, these GBOs are invaluable because, in addition to just maintaining the diverse heterogeneity of cell types from the parental tumor, we can map over transcriptomics from the parental tumor through a 2D culture of becoming the GBOs that a lot of the original cell clusters are maintained. As seen here in the maps, these correlate very strongly to the original tumor signifiers that from which they came.
What we wanted to do was to see if we could take these GBOs from our patients in this trial and, if we took some of the autologous patient CAR T-cell product that we are testing, we can perform in vitro assays that would give us any more information on how the patients are responding in real-time.
As you can see with the CAR T-cell trial, these patients are getting a multitude of assessments performed such as radiographics. We're drawing CSF from these patients in order to look at CAR T-cell expansion and cytokines present in the in the CSF. On the GBO side, after they've received surgery and we were able to generate their GBOs as well as the patient product is manufactured, we were able to take those two things and co-culture them. Then, we were able to take a look at parameters such as tumor cytolysis, CAR T-cell activation, engraftment, and cytokine expression in the media of these assays to look and see if we can deduce if they even look similar to what's happening in the patient.
Again, these assays that are going to be described here are going to include GBO and autologous CAR T-cell products, impedance assays, cytokine analysis from these co-culture impedance assays, immunohistochemistry of cultured GBOs retrieved from impedance assays, as well as flow cytometry of the GBOs and CAR T-cell products retrieved from these assays. Why this was really important for us to do is that it could be done in real-time alongside the patient treatment.
What that actually looks like in terms of a physical timeline is that the surgical resection takes place, and then the CAR T-cell treatment has begun within about a month of that surgical resection. At Day 0 of the time of treatment, we have already produced the GBOs and received the excess clinical product so that we could start their co-cultures alongside patient treatment timeline. Since the GBO co-cultures that are performed run about six days long, and our first patient MRI isn't even until Day 28, we're actually seeing evidence of cytolysis by the patient product within a fraction of the time it takes for us to see our first patient MRI.
The real work horse of these assays is the impedance platform which we partnered with Axion Biosystems. We used a Maestro ZHT impedance system to look at, and I cite their application note here—which is what gave me the original idea to perform these real-time assays in comparison to a chromium release or something else that was more of an end-point assay—because we wanted the real-time output. They had designed protocols whereby you could do this on spheroids in their CytoView Z 96-well plates as opposed to a 2D monolayer cultures.
What we can see is that they show that you can have different densities of spheroids. Added to their plates, they create an impedance or resistance signature that we can then add effector T-cells, which affects that resistance and can be converted into cytolysis outputs.
What I did was based on this application note. I took GBOs, plated them into PDL- and laminin-coated CytoView Z 96-well plates and allowed them to adhere for 24 hours before adding effector CAR T-cells. I separated these into one GBO per well. They were rather large and they come out to be around 100- to 200,000 cells per organoid at the time of placement.
These are pretty large and cover a decent bit of the surface of the CytoView Z plates and create a nice, robust resistance signature. Then, we add the autologous CTI cells at a 1:10 effector-to-target ratio. These cultures were monitored for six days before cells were retrieved. This includes overtime at relevant time points. I did pull out media from these assays to do cytokine profiles.
First, looking at what these GBOs looked like, I’m showing you here data for six patients from the trial. We show that from all six patients, we were able to isolate the GBOs. When we look at the patient GBOs before starting, we had to validate that the target antigens were present in the GBOs and maintained from the parental tumor.
As a side note, in the clinical trial enrollment criteria only included the amplification of EGFRvIII. We did not have to analyize the IL-13Rα2 signature. This was also kind of a "sanity check" for us to make sure that both antigens were present in these GBOs and in the parental tumor.
For each patient, on the left, you see immunohistochemistry (IHC) images showing DAPI, EGFRvIII, IL-13Rα2, and a merge of the post-resection tissue before processing into GBOs. After 21 days of processing into GBOs, all you need to takeaway from this slide is that we have nice, robust presence of both target antigens in every single GBO. Thereby, we know that when we introduce these into our co-cultures there should be targets for these CTI cells to find.
As we move into the data from the impedance co-cultures, what we can see is that from our first six patients is if you take their GBOs and co-culture them with their matched autologous patient product, they all have differing levels of cytolysis over time. That is significantly different from untreated GBOs in all six patients, so there were levels of cell killing present.
Across each patient, there were obviously different amounts of cytolysis in terms of a different response, which was to be expected in terms of patient-to-patient variability. This becomes more important as we move into what's actually happening in vivo.
If we look at the same six patients, the data on CAR expansion—published earlier this year in our group’s Nature Medicine paper again on the first six patients—shows that we probed the CSF to look at the amount of CAR copies per microgram of genomic DNA present. This was to ensure that the CAR T cells were actually expanding in the CSF and were present. What we saw is that there was a huge spike within the first day or two after infusion, leading to this maintenance or a slight drop-off in the amount of CAR copies found in the CSF.
This adds a lot more color to why we some differing levels of cytolysis. One of the really interesting findings from this study is that, when we look at the percent cytolysis observed in the first 24 hours of the impedance assays, it strongly correlates with the peaking engraftment data from these same six patients. This could be an early indicator. Even though we saw what seemed to be very variable responses, the impedance data actually correlates very strongly with the amount of CAR expansion observed in the short-term. It gives us a bit more insight into how robust the CAR expansion was across those patients.
To ensure that what we were seeing was on-target killing, we orthogonally confirmed this by analyzing GBOs out of those impedance assays. We did cleave caspase-3 immunostaining after six days of co-culture. What you’re seeing here is that between untreated and treated conditions for each patient (one through six), we saw this massive expansion of CD3+ T cells, as well as the presence of cleaved CPAs 3, which is an apoptotic marker.
This is showing that the CD3+ T cells we are incubating with these GBOs are not only invading into the GBO but also causing cellular apoptosis as a result. This was confirmed to be significant across all of our patients at the endpoint.
To confirm that these cytotoxic events were due to on-target antigen recognition—and not off-target toxicity or native TCR recognition by the patient product—we performed a couple control experiments using an off-trial patient GBO. This patient received their surgical resection but unfortunately had to be removed from the trial for other medical reasons. We received their GBOs and their patient product and conducted impedance assays, where we looked at them over the six days with untreated, their autologous matched product, a CD19 off-target CAR made from their autologous T cells, and un-transduced autologous T cells.
What we can see at the endpoint was a significant difference between the autologous product containing the on-target CAR versus the off-target CD19 CAR or un-transduced cells. This was also reflected in the cleaved caspase-3 confirmation.
The fact that we also saw no significant Ki-67 content in the CD3+ cells found in GBOs that received un-transduced T-cell treatment, means that none of the T cells in those GBOs were expanding as a result of activation.
I think possibly the most impactful piece of data from this control experiment was that in GBOs treated with the on-target CAR, off-target CAR, un-transduced T cells, or even in the T-cell-only cultures, none of them created a cytokine expression profile that included interferon-gamma, IL-2, and TNF-alpha unless it was the on-target CAR with the GBO. The off-target CARs were not contributing to any cell killing and therefore were not releasing any cytokines.
To further elaborate on the on-target activity, we also performed IF to look at the amount of the actual antigen present in the GBOs before and after treatment. What you’re seeing here is that for each patient, we have untreated and treated GBOs with the presence of the EGFR or IL-13 receptor alpha-2 antigen shown, respectively. What we observe across almost all of the patients is that there are significant reductions in the amount of EGFR as well as IL-13 receptor alpha-2 post six days of treatment.
In addition to IF, we also wanted to examine the double-positive and double-negative populations. This is really impactful because when we pull the GBOs back out from these co-cultures at six days, there are still a large amount of cells there. These are the cells we would assume, in vivo, are leading to recurrence because they are either avoiding detection, avoiding destruction, or are double-negative from either of the antigens present on our CAR T cells.
What we did in order to look at more complex populations was we took the GBOs out from the impedance assays after six days and performed flow cytometry. We could really look at those double-positive, single-positive, and double-negative populations. What we see for each patient—and I’m going to look at the summary data here—is that for the double-positive cells after treatment, there is a significant reduction in all of the double-positive cells, which is what we would expect. There is a significant increase in the double-negative population.
These are the cells that would theoretically be escaping treatment, as well as a large amount driving the EGFR-positive single-positive populations down. Interestingly, we did see an increase in the IL-13 receptor alpha-2 single-positive populations.
In conjunction with other data we’re pulling from both patient data and other data not shown here (but available in the paper you can find on bioRxiv), we think that a lot of the CAR activity is being driven more by the EGFR than by the IL-13 receptor alpha-2. This is still being studied and will continue to be studied on what the changes in these single- and double-positive populates mean as we increase our patient numbers and the number of GBO assays we’re doing.
Lastly, one of the coolest and most impactful pieces of data we got from the patient impedance co-cultures is that when you take media from these co-cultures, they exhibited commensurate trends in cytokines, as seen in ELISA, after CTI cell treatment. These trends mirror what we see in patients.
What you’re seeing in Panel A is that for each patient and each cytokine, the GBO spikes and then plateaus or decreases in the amount of cytokine present directly mirrors what was observed in the patient CSF values—this is for almost all six patients. The fact that not only are the trends mirroring but also the actual concentrations of cytokines in the wells compared to the CSF being commensurate was pretty incredible to see—especially when you look at Panel B.
When we look at these three key cytokines over time in the GBOs versus the co-culture, a lot of these were trending very similarly. Lastly, there's not many that are significantly different from each other over these first six days. These cytokine releases happening in the plate are very similar to the cytokine release happening in the patient CSF after treatment. This could serve as a good additional real-time assessment to give you more insight into what’s happening in the patient.
To look at this from another way, what we see here is the data from our GBO study here compared to the data published in the Nature Medicine paper from the patients where they look at INF-gamma, IL-2, TNF-alpha, and the inclusion of IL-6, which we hope to include in future works. The amount of cytokines and the peaks present all change the most within the first seven days—a critical window of looking at bioactivity. In the patient, they seem to plateau within the first month.
Ultimately, since we know we can take these GBOs out a little longer, I think this will likely be included in future studies to see if we can maintain that kind of high degree of fidelity between the two over a longer period of time. This could get more information from the GBOs, but this remains to be looked at.
A couple of conclusions from this work, the GBOs that we generated from our patient resection tissue can be used with high fidelity to represent certain features of patient GBM. It’s not perfect, and it’s not meant to be, but it works us one step closer towards looking at ex vivo biomarkers of what's happening in real-time.
The ex vivo cytolysis data generated here from the Maestro ZHT was highly correlative with patient response to treatment during the early critical time points of bioactivity. We also saw that the ex vivo assays performed here in the CytoView Z plates had very proportionate cytokine dynamics as the in vivo patient CSF at the same time points. This will be critical as we move forward, studying more patients and hopefully examining longer time points to see if we can learn even more information from these ex vivo assays. These insights will help us understand recurrence dynamics or responder dynamics in these patients.
Lastly, some immediate future work we plan on doing to expand our patient cohort to gather more data and exploring dose ranges. One particularly interesting things that we didn’t look at is that we didn't change our effector-to-target ratios throughout these assays. One of the things in the future would be changing up is the effector-to-target ratios to see if we can better mirror patient dosing dynamics in these killing assays.
Other immediate future work is that, like I mentioned, when we look at the antigen presence before and after treatment is that those populations that are left over after six days of treatment. Especially because those are the cells that are left over after that critical first week of bioactivity of the CAR in the patient; how they contribute to recurrence. We can use rechallenge assays in the Axion impedance platforms to look at how those resistant populations survive and maybe how we can start trying to target those better.
With that, I’d really love to thank my lab and everyone who contributed to this work. They made it so much fun. I’d also like to thank our collaborators in the Song lab, who were critical in harvesting and maintaining GBOs, as well as in data collection and analysis. I’d like to thank anyone who worked on the clinical side with the clinical trial, including Steve Bagley, who is the PI for this ongoing Phase 1 trial at Penn. Special thanks to Axion BioSystems for their collaboration in helping with instrumentation and experimental design, as well as our funding sources.
Thank you for having me here today to talk about this work, and I’d love to take any questions you might have.
Lauren Coyle:
Thank you, Meghan, for that absolutely fantastic presentation. As we mentioned, we will now be starting our Q&A session, so please keep your questions coming in. Meghan, if you'd like to join me on camera—wonderful, thank you for being here.
We’ll just kick off with our first question: "How long can these organoids be maintained ex vivo until they no longer represent patient tissue?" Oh, I think you might be on mute there.
Meghan Logun:
Sorry, I was—thank you. I was saying that's a great question, especially because I know a lot of other people studying cancer tissues have the same problem.
Right now, I would say that we have data showing that key critical aspects are maintained through 12 weeks of active culture. Then, GBOs that have been biobanked before that point—frozen down and then thawed well beyond a year—have been shown to retain many of those same key features that we look at.
As we continue to expand our understanding, I imagine we’ll start looking at even longer time points, like 24 weeks or three-month-long data points, at some point in the future.
Lauren Coyle:
Wonderful, thank you so much. Our next question here is: "Did you dissociate the GBO into a smaller size for the impedance assay?"
Meghan Logun:
So, for the impedance assay—no, they were not dissociated. What we found was that the GBOs were large enough to actually, when placed on those coated plates, form adhesions across the bottom of the GBO. They had an impeded signature that was large enough to then look at changes when we added the effectors.
We did, however, dissociate the GBOs specifically for flow cytometry since that is more of a single-cell-based analysis.
Lauren Coyle:
Amazing, that was great, thank you. You mentioned the hypoxic environment of the TME: "Do you ever look at the T-cell phenotype of your CTE cells before and after being co-cultured with the GBO, and what impact do you think the hypoxia had on efficacy in your model?"
Meghan Logun:
Yeah, that's a great question. So, we do have the data for the T-cell phenotyping of the CAR patient product for all the patients. I didn’t go into it here due to the limited time, but I definitely recommend checking out the Nature Medicine paper if you’re interested in that, as well as this other paper—which we just received word was accepted and should hopefully be out soon.
As far as what impact I think it had, I don’t have a good answer right now based on what we’ve seen because we didn’t specifically look at hypoxia in this study. But, I know that in future studies, we will more deeply look into the CD3-positive T-cells that penetrated into the organoid. We'll examine the depth of penetration and its effect on T-cell phenotype and persistence, as well as possibly putting the GBOs in hypoxic chambers and doing co-cultures within the hypoxia chambers to bring the microenvironment into play.
Lauren Coyle:
Amazing, thank you. Can you elaborate on some of the differences in using this platform between 2D and 3D?
Meghan Logun:
Yeah, I think that’s a great area of comparison. I think that here we really wanted to do here is, we know—and have a lot of data—on our CAR killing in the 2D adherent substrate models. What we really wanted to do here was look at the difference that the 3D microenvironment made in terms of if we could measure killing in a bulk environment where the T-cells really have to work for it, compared to just laying them over a 2D platform. Since the 3D environment is more realistic to the in vivo setting.
Lauren Coyle:
Wonderful, thank you. Do CAR T-cells exert killing effect only when two targets—the IL-13Rα2 and EGFR—are bound?
Meghan Logun:
Yeah, that’s a great question, and the short answer is: almost always. We have data from previous papers showing that off-target toxicity for these two targets specifically is low. If you remember, I mentioned the EGFR target—we’re not binding wild-type, we're binding a specific tumor-associated mutation. With IL-13Rα2, it’s primarily prevalent in that brain tissue. So, we see very little off-target toxicity in our assays that we do in other platforms.
Lauren Coyle:
Wonderful. We have time for probably just one more here: "In the future, if a patient’s GBOs did not respond to CAR T therapy in vitro, what could a clinician do with this information? Could they plan to add additional treatments or perform further function or genetic testing on restraint cells to find therapeutic targets?"
Meghan Logun:
Yeah, that's a great question. I think it goes straight to the heart of the ultimate vision for these as both predictive medicine tools as well as establishing some of those mechanistic wise of why we see responders and why we don't. Ideally, what we’d really love is for these to be used, as you said, to give a clinician a bit more insight into what’s going on so they can determine: "Okay, is this patient is a prime eligible or a prime candidate for redosing, are they a prime candidate for maybe increasing the dose, or not should we discontinue and try a different trial that might be a better use of the patient’s time?" Looking at those resistant populations could help figure out why this patient isn’t responding to treatment.
Lauren Coyle:
Fantastic. Well, Meghan, thank you so much for answering all of those questions. But, that is all the time we have for today so any questions we didn’t get to we will reply to by email.
The webinar will be available on-demand tomorrow, so look out for an email from us with the link. We’d also like to invite you, the audience, to take a short survey to provide us with feedback on today’s webinar. The link can be found in the resources on the right-hand side of the screen or in the on-demand email tomorrow.
So, all that’s left is to thank Meghan once more for a great presentation, and thank you all for listening! We hope you’ll join us again soon.