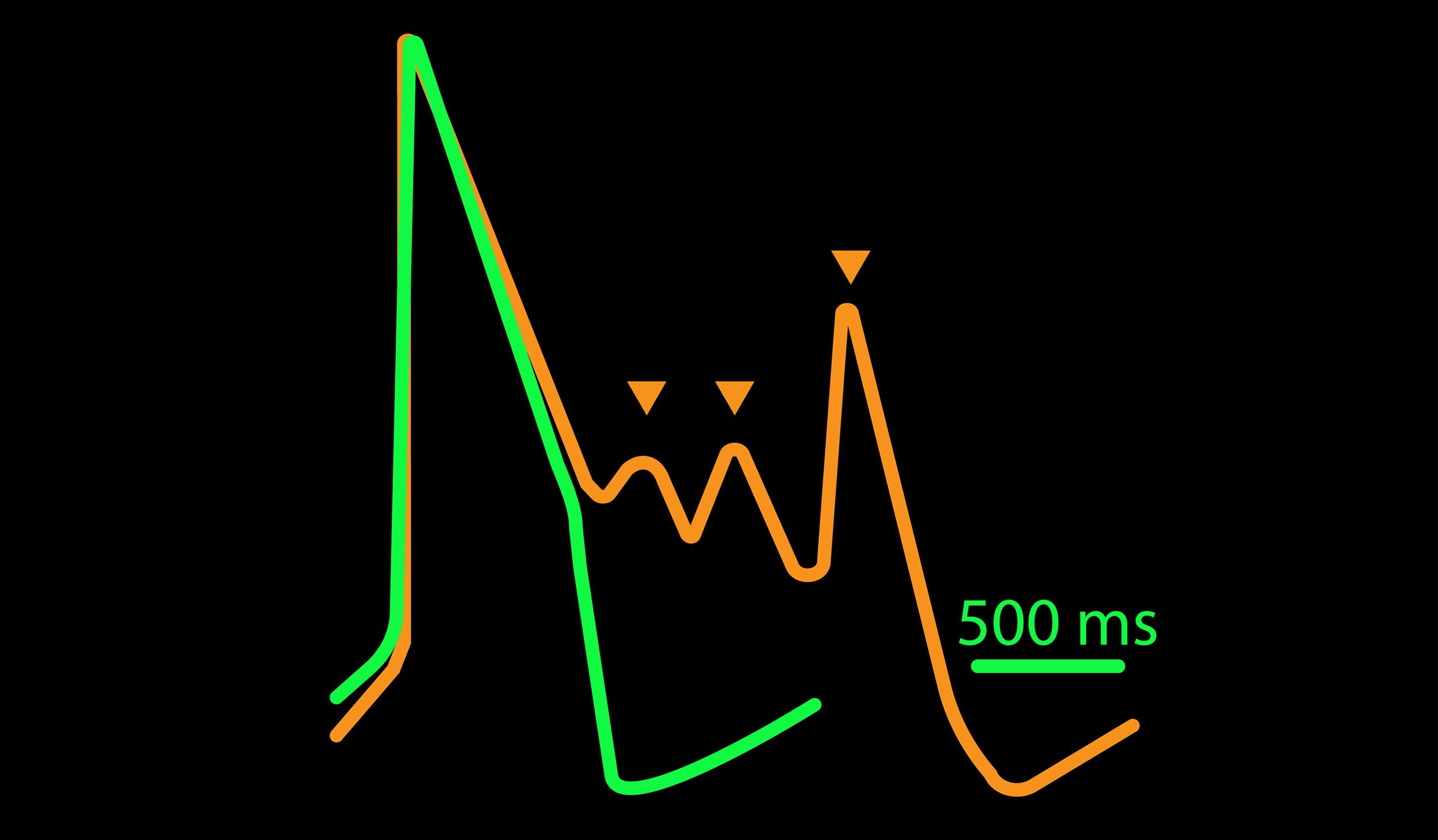
心毒性は、自然及び人工の物質によって引き起こされる心臓の機能障害や損傷を意味します。創薬において、臨床試験前の段階で治療化合物の心毒性を予見することは極めて重要です。
Maestro MEA は、in vitroでの心毒性評価に広く使用されています。プレート上に埋め込まれた複数の平面微小電極を用いて心筋細胞の電気的な活動を測定します。複数電極による測定は、心筋細胞のシグナル伝播の評価にも最適です。また、同一電極を用いての弛緩収縮の評価も可能で、1度のアッセイで多くの情報が得られます。
心筋細胞を用いた安全性試験
The Comprehensive in vitro Proarrhythmia Assay (CiPA) は新薬の催不整脈リスク評価系の改善を目的としてその活動が行われています。CiPA パイロットスタディでは6施設がMaestroを使用し、2種類のiPS細胞由来心筋細胞を用いて8種類の既知化合物の評価を行いました。Maestroによる薬剤応答の変化の検出は正確で、また、施設間及び異なる細胞種において高い再現性が得られました。
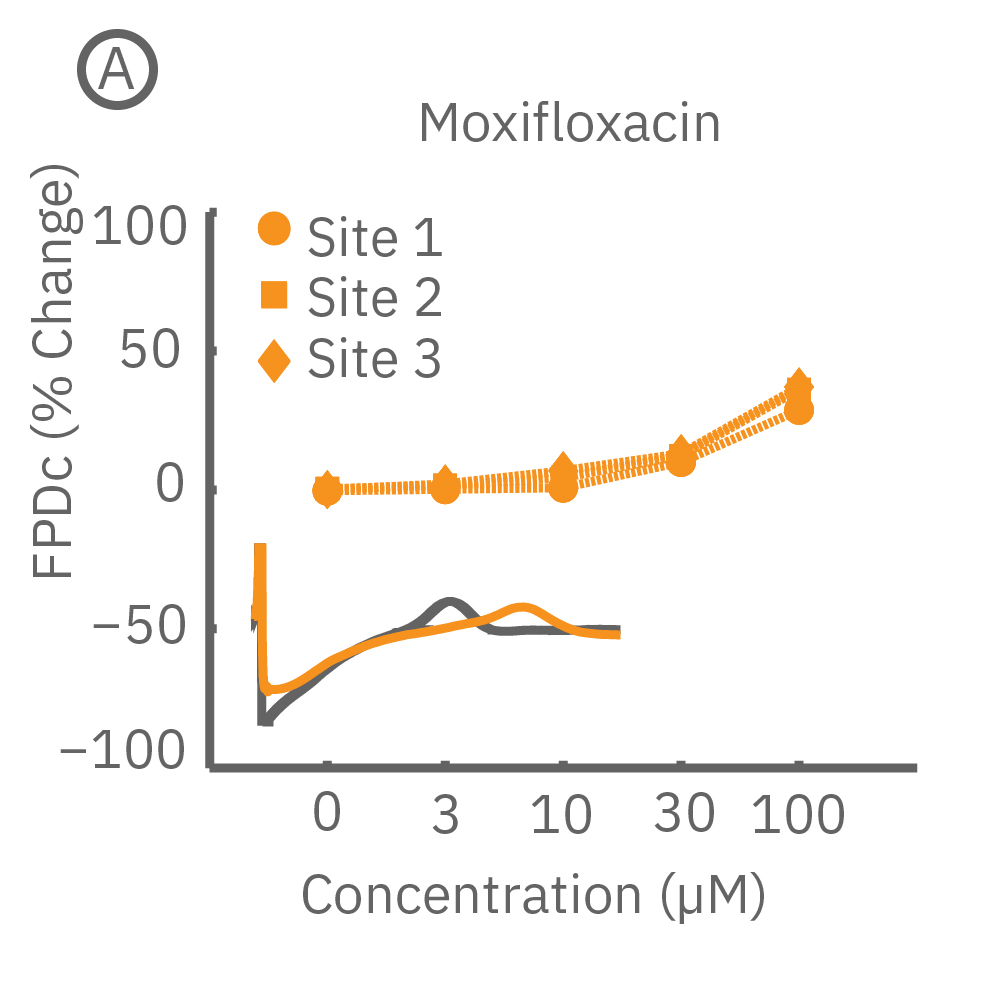
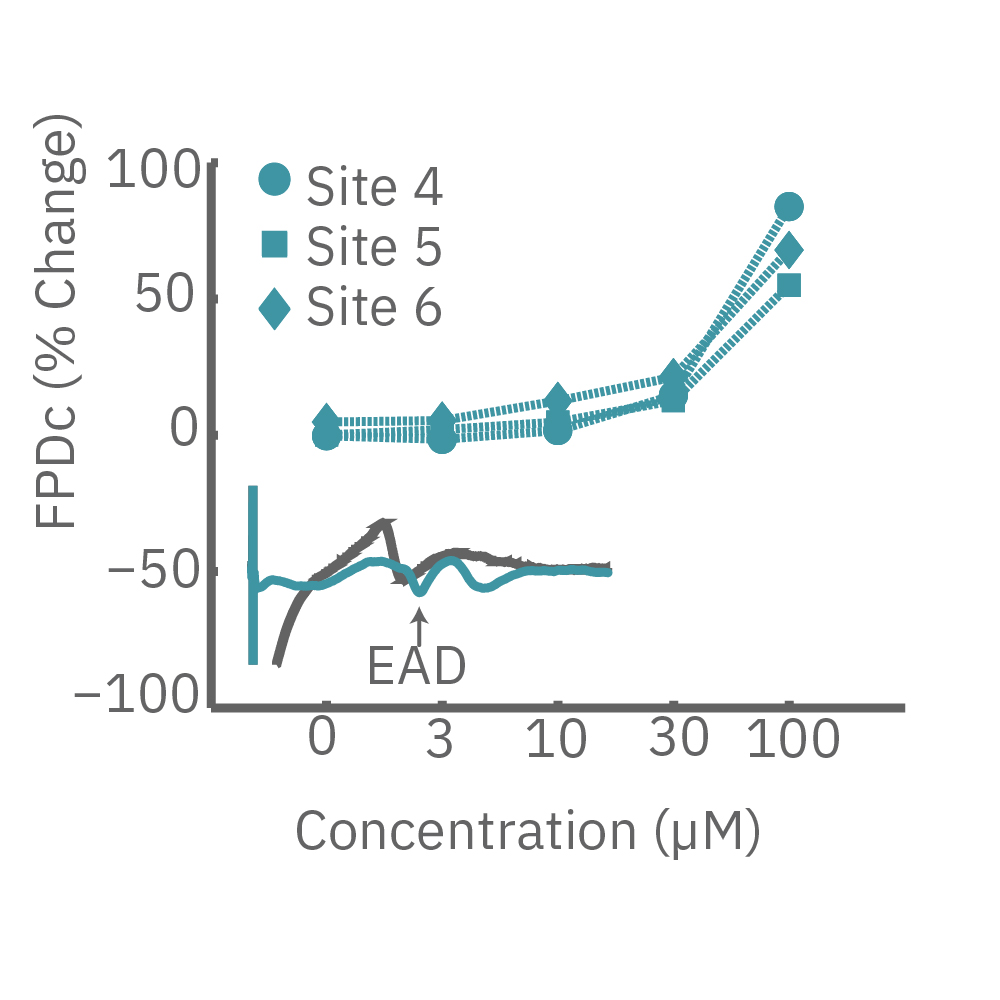
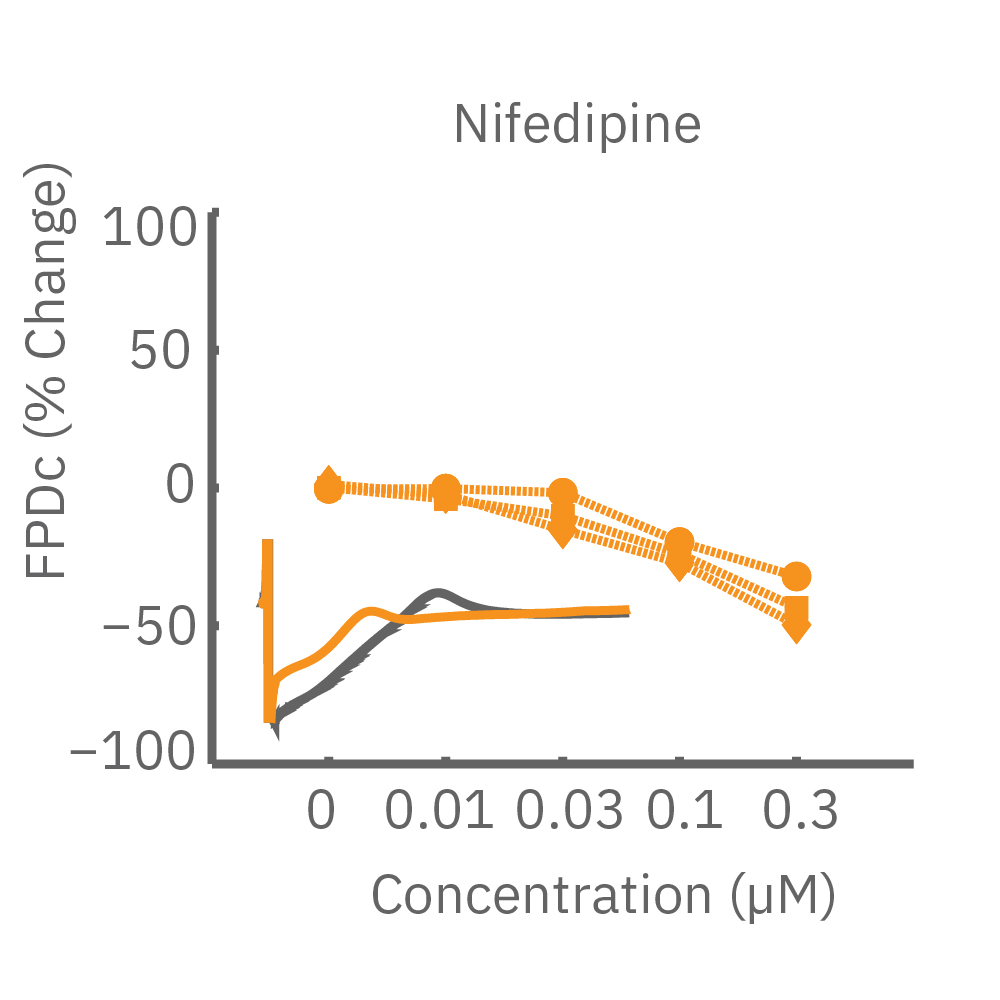
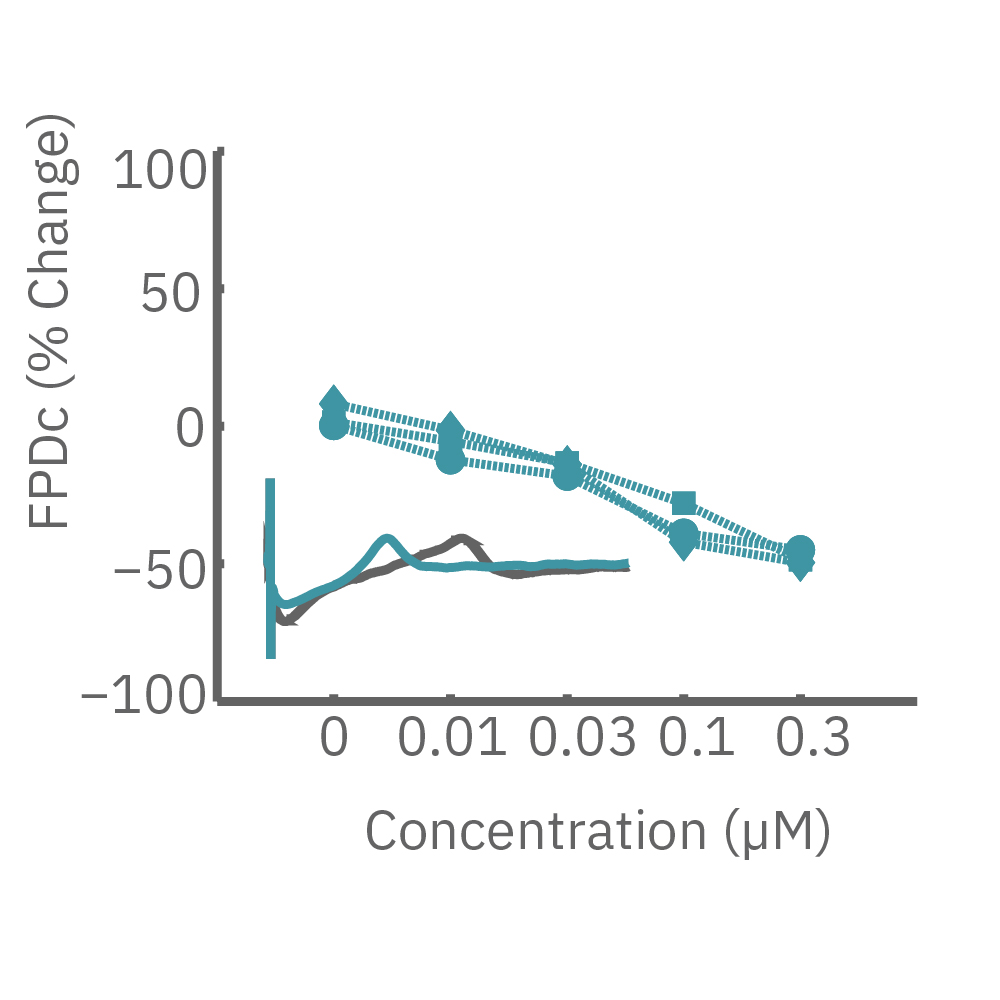
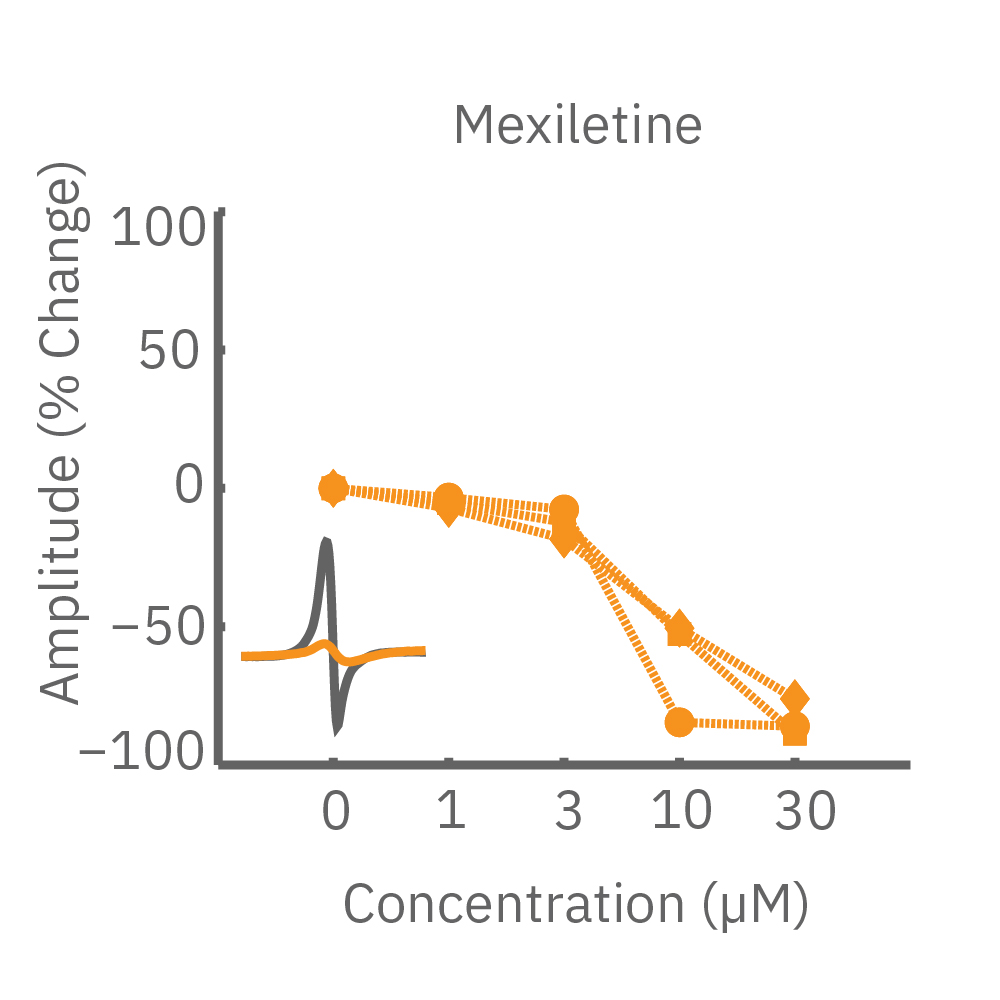
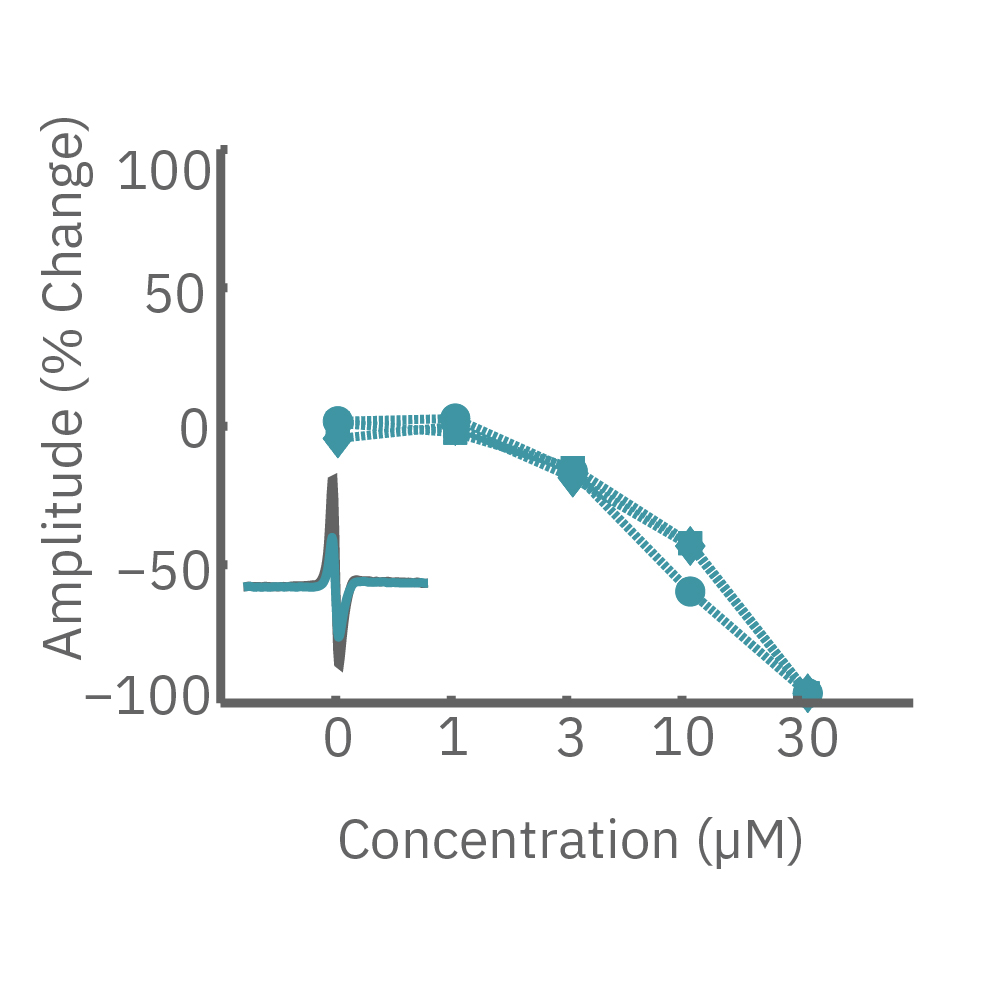
CiPA パイロットスタディにおける Maestro 測定結果の一部。(A) は6施設よる、2種類のiPS細胞由来心筋細胞 (Cor4 U/オレンジ (Axiogenesis社)・ iCell/水色 (CDI社)) を用いた3種類の化合物評価の結果を示す。
Moxifloxacin (hERG K+ 阻害剤) の投与でFPD (Field Potential Duration) の延長(左)、Nifedipine (Ca2+ 阻害剤) の投与でFPDの縮小 (中央) が得られ、Mexiletine (Na+阻害剤) の投与ではスパイク振幅値の減少(右) が得られた。また、同様の結果が複数施設、2種類の心筋細胞に渡り再現された。
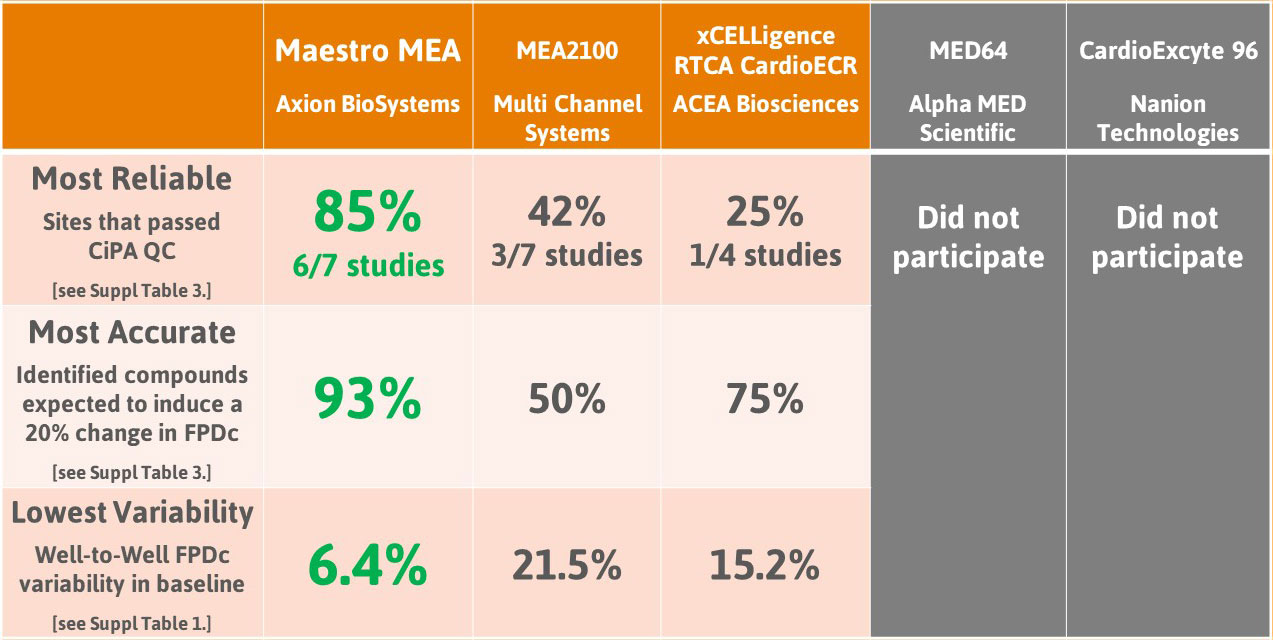
複数施設による未知化合物の評価を行ったCiPA パイロットスタディでは、iPS細胞由来心筋細胞が、心臓に影響を及ぼす化合物の検出に有用であることが実証されました。Axion BioSystems は本スタディで重要な役割を担ったことを誇りに思い、また参加された Maestro ユーザー様に感謝いたします。
Download CiPA Pilot Study Paper
パイロットスタディに続いて実施された CiPA Myocyte Phase II validation study では、先の Phase I スタディの領域を拡大し、低・中・高の TdP (torsades de pointes) リスクを有する28 の薬剤を11施設にて評価しました。本 スタディでは、iPS細胞由来心筋細胞が、薬剤による不整脈反応の検出において有用であることが実証されました。Axion BioSystems は本スタディにおいてもリーディング的な役割を担ったことを誇りに思い、参加された Maestro ユーザー様に深く感謝いたします。
Download CiPA Phase II Study Paper
LEAPによるヒトiPS細胞由来心筋細胞を用いた薬理試験
LEAP (Local extracellular action potential) は、Maestro Pro/Edge の特有機能です。プレート底面の平面電極を用いて、Action Potential 形態シグナルを測定します。EAD (early after depolarization) などがよりクリアに検出されます。薬剤投与などにおいては、パッチクランプのシグナル変化と同様の変化を示します。
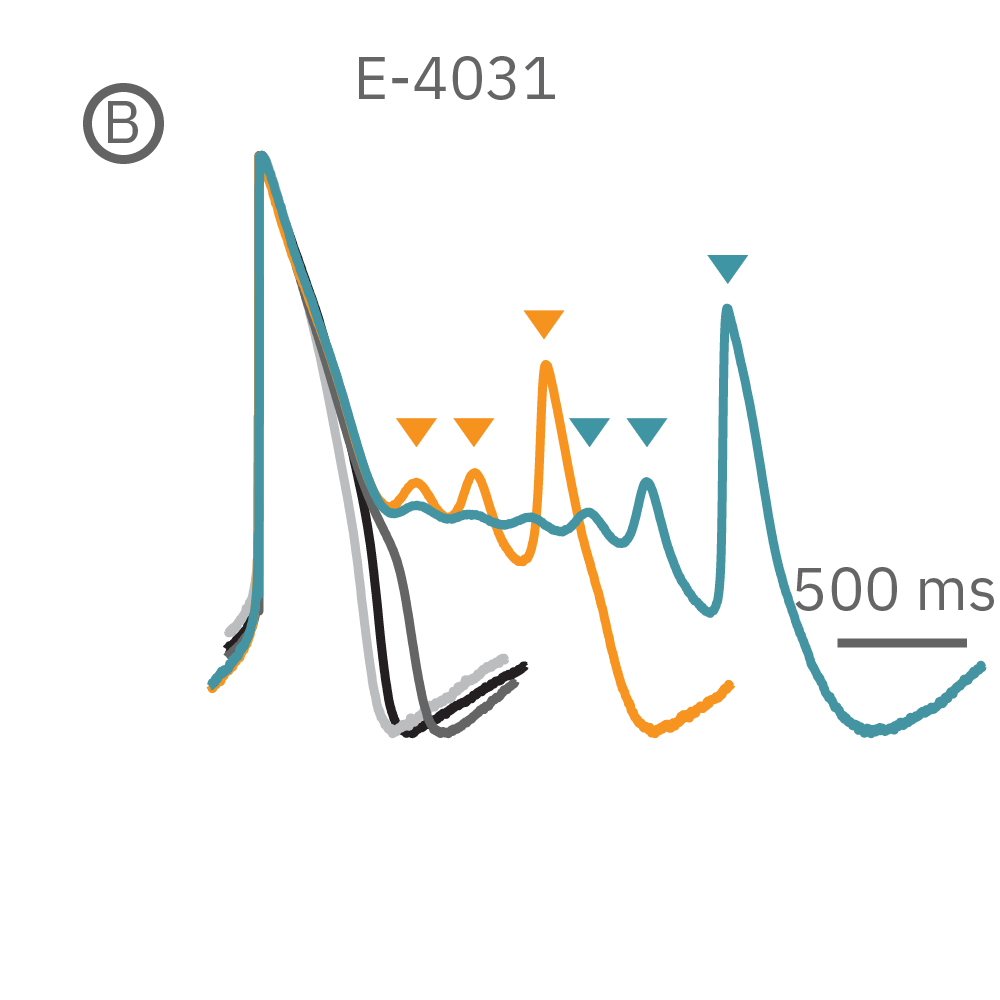
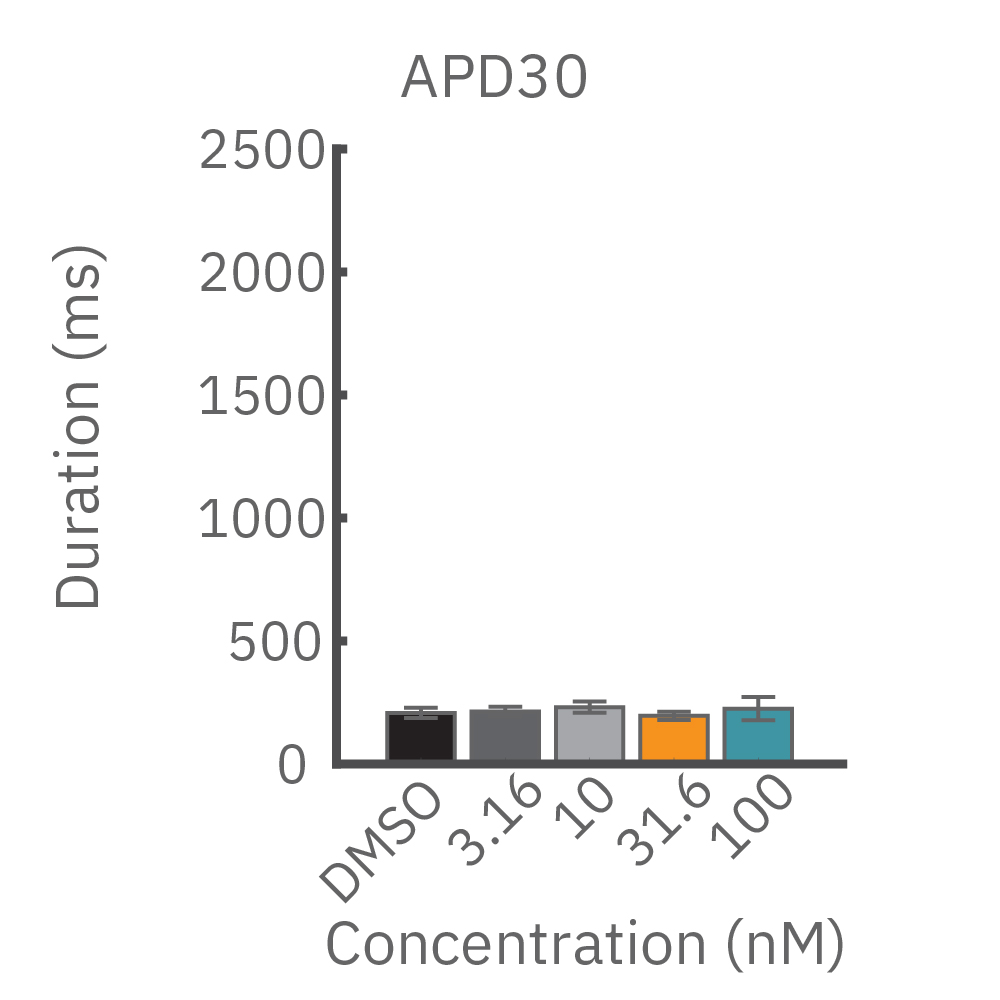
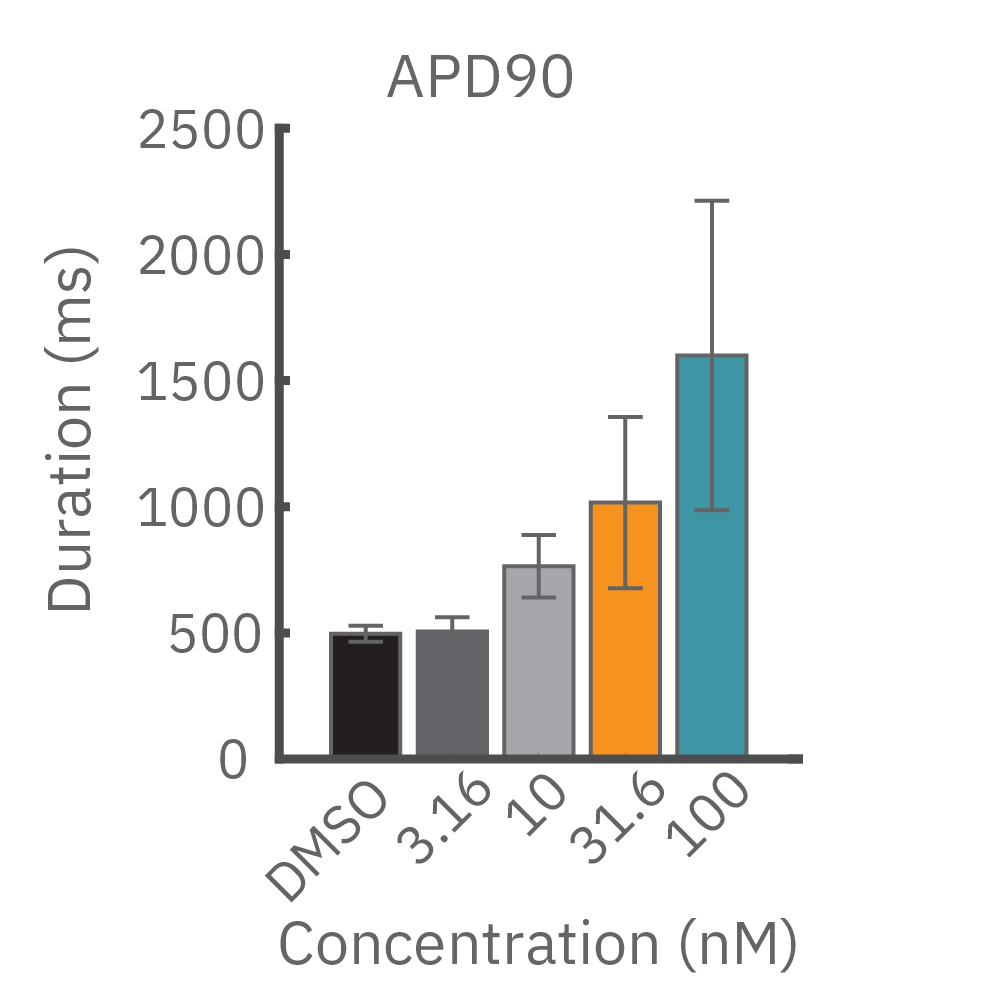
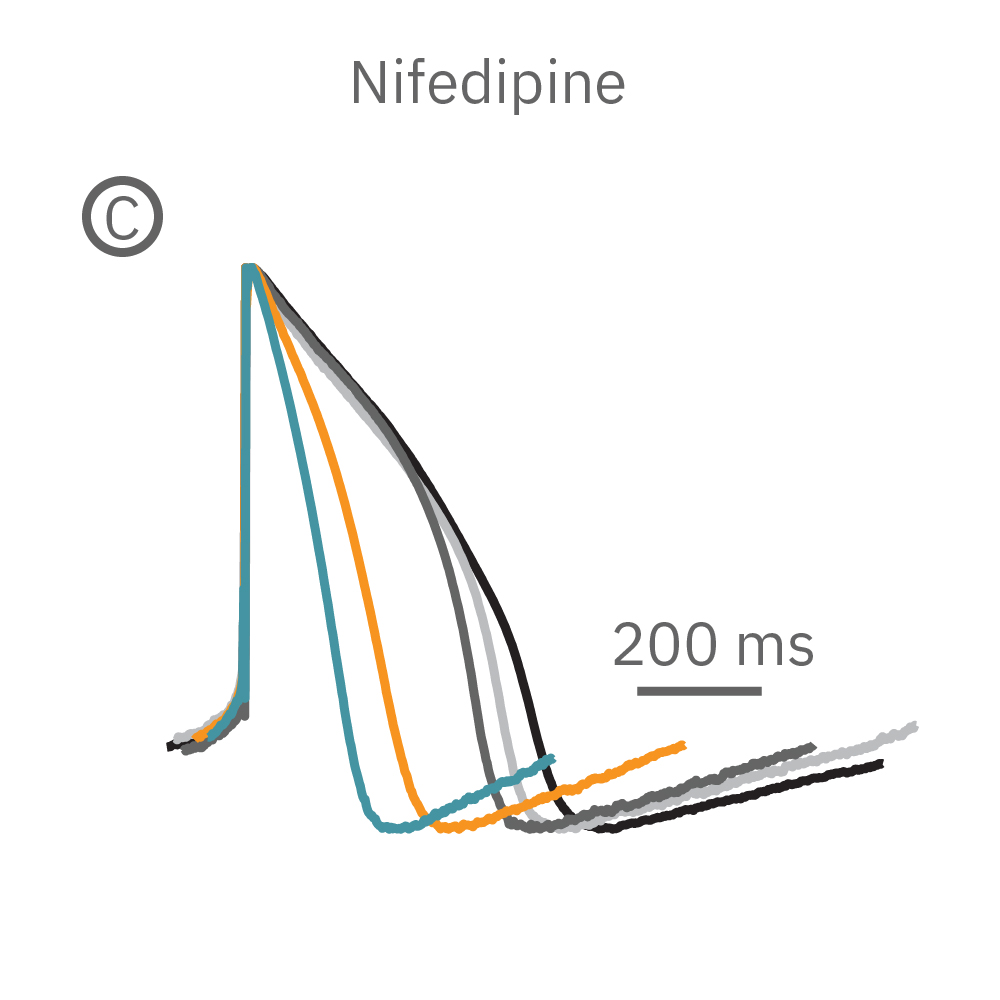
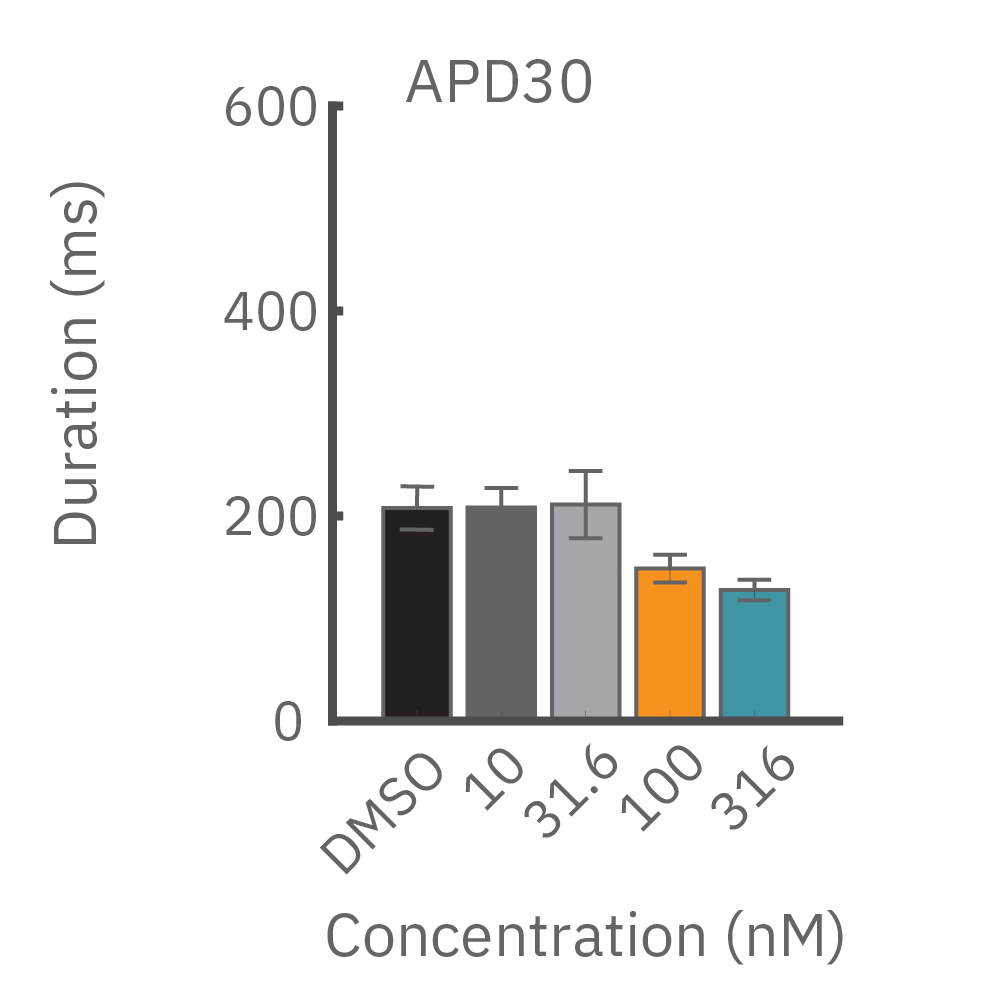
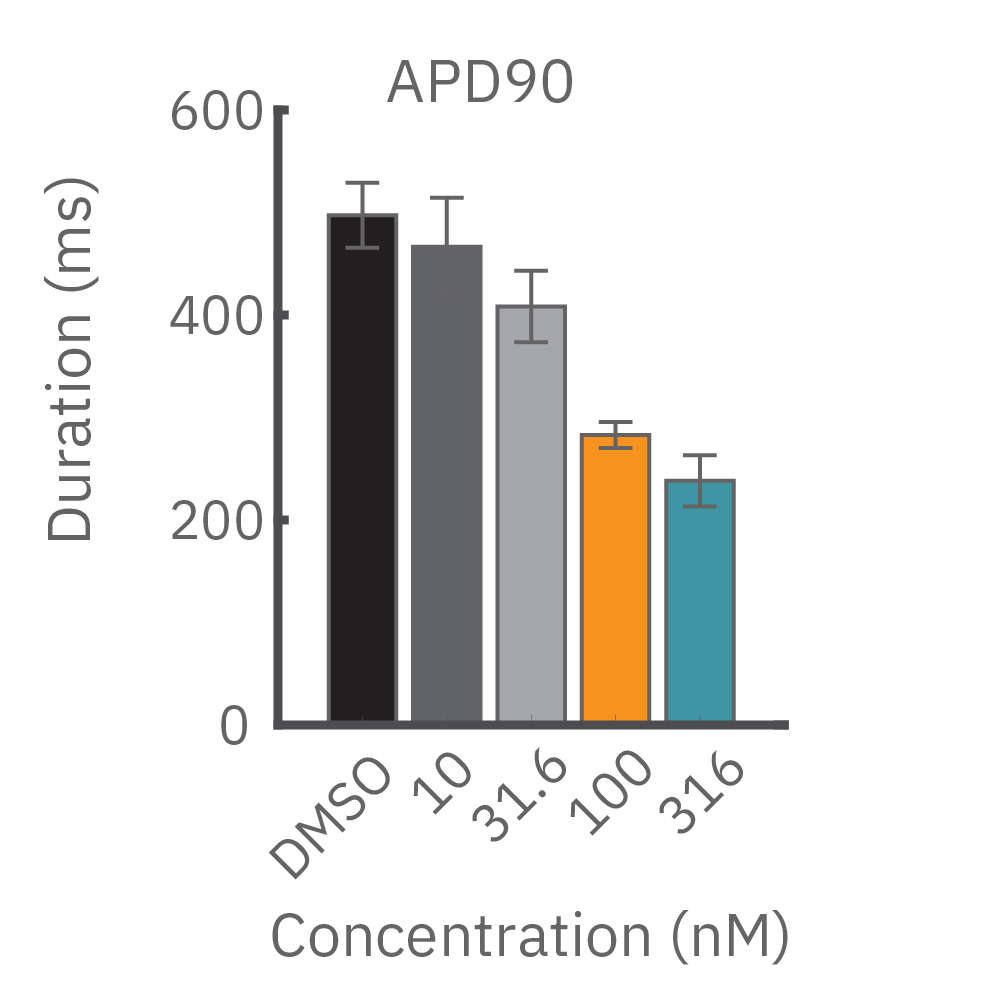
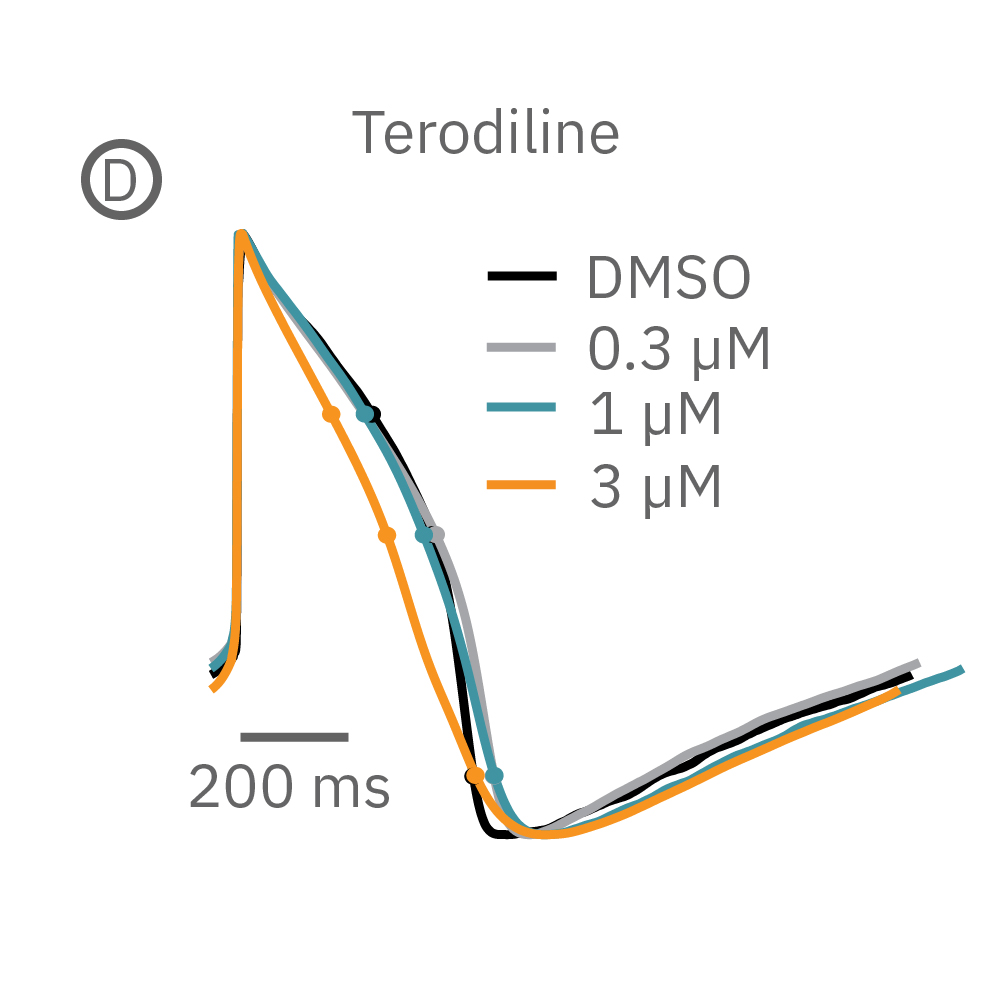
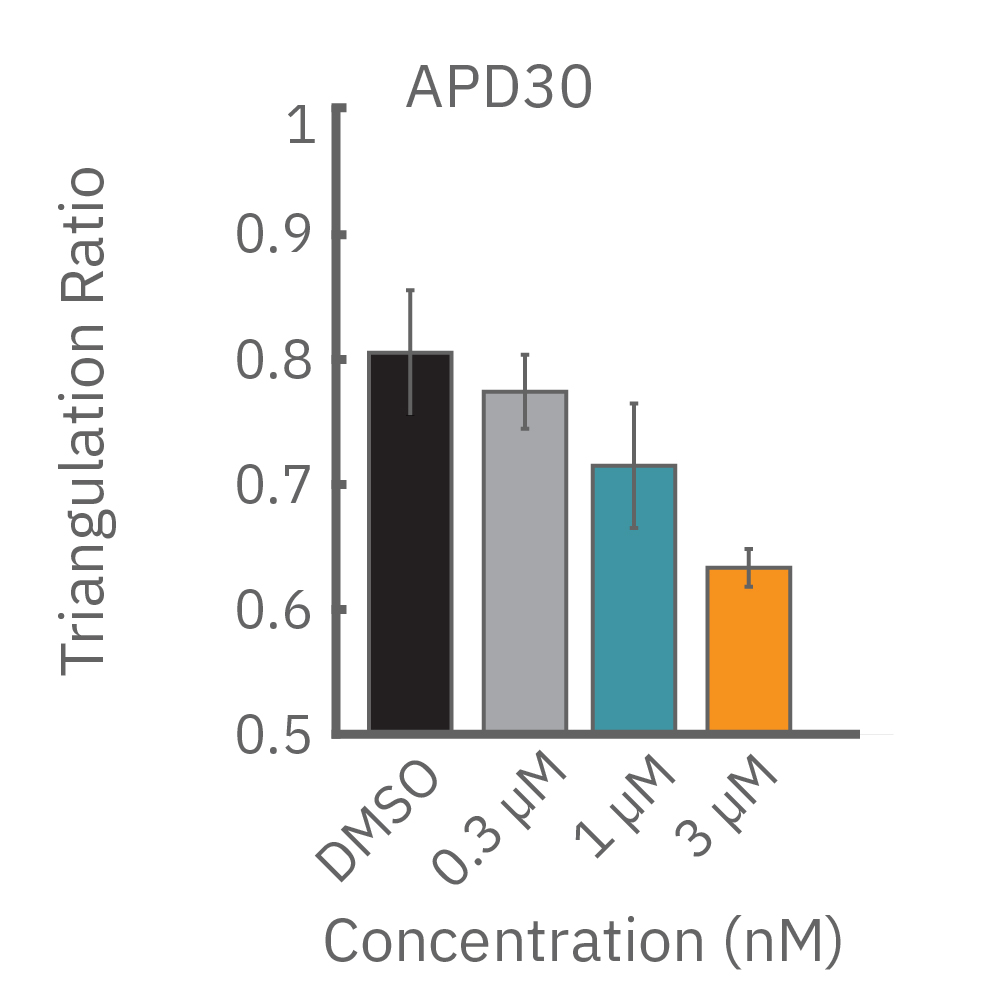
(B) E4031 投与による LEAP シグナルの変化。再分極フェーズの遅延と、EADを示唆する非規則性が得られた。
(C) Nifedipine 投与による変化。LEAP シグナルの持続時間は濃度依存的にに縮小した。
(D) Terodiline 投与による変化。高濃度化において三角形化 (triangulation) が見られ、パッチクランプデータとの相関が得られた。
化合物による伝播パターン及び伝播速度の変化の検証
iPS細胞由来心筋細胞合胞体においては、拍動は培養内の1点で発生し (pacer region)、培養内で波のように伝播します。薬剤や心疾患は、細胞の興奮性や細胞間のギャップ結合を改変させ、この伝播に影響を及ぼすことがあります。Maestro MEAによる複数電極からの電位応答測定は、これらの検証に最適です。
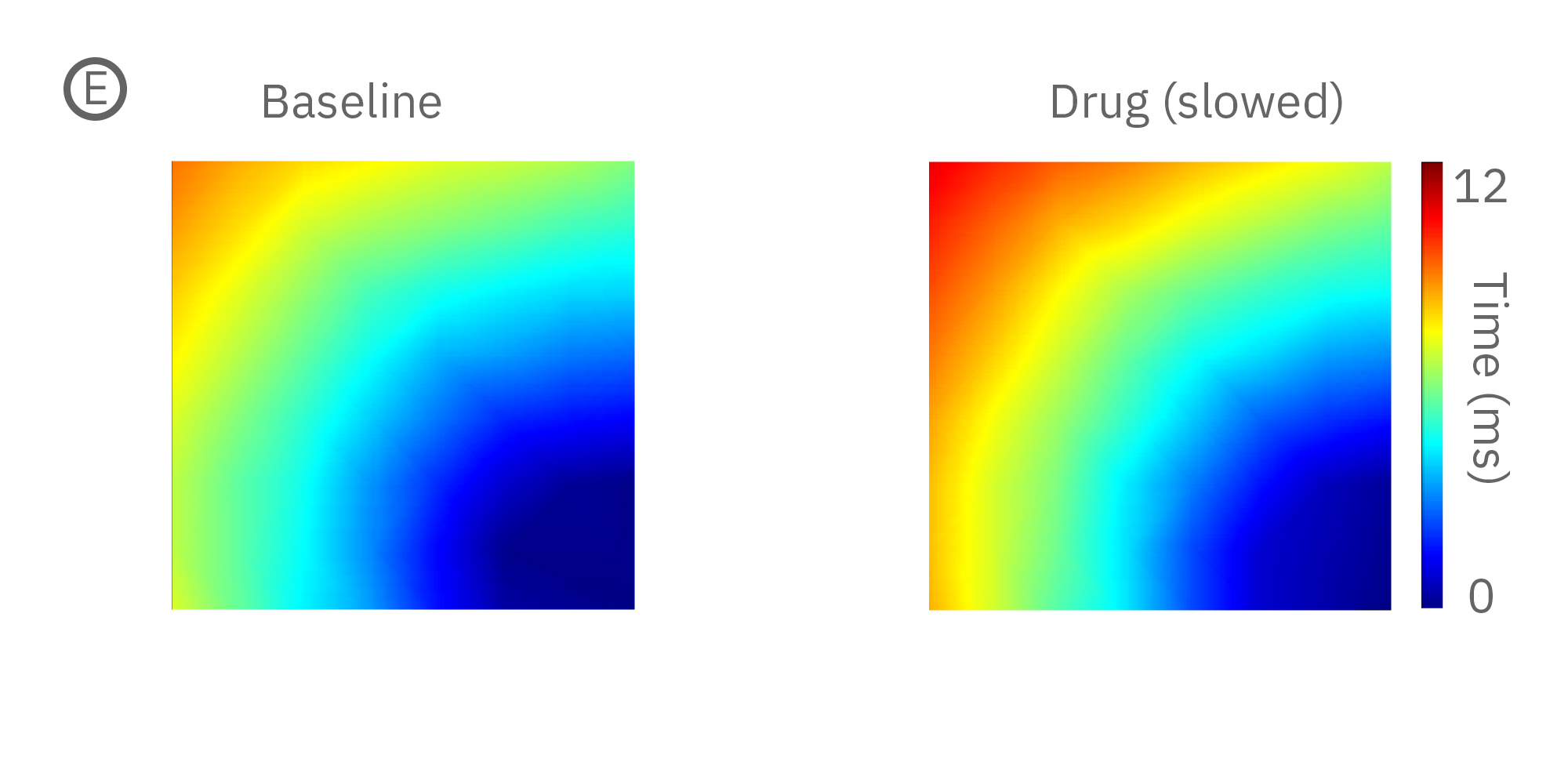
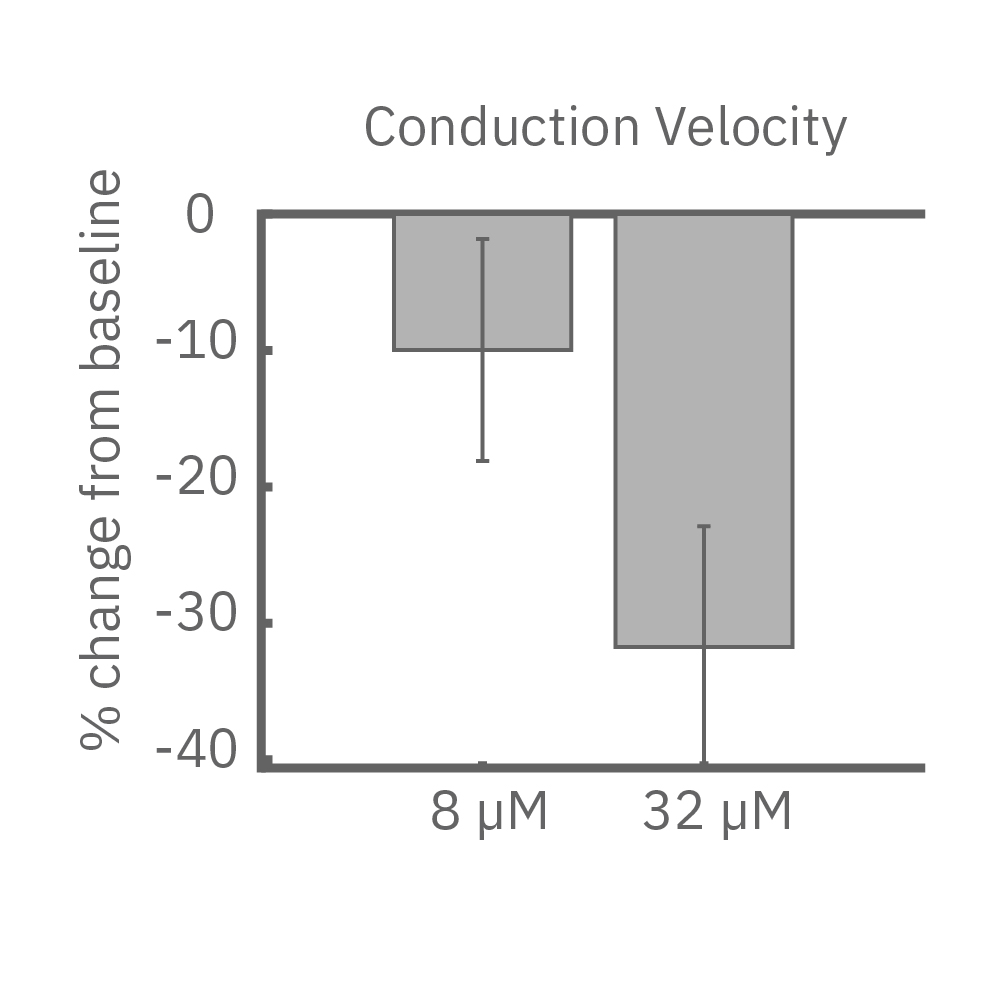
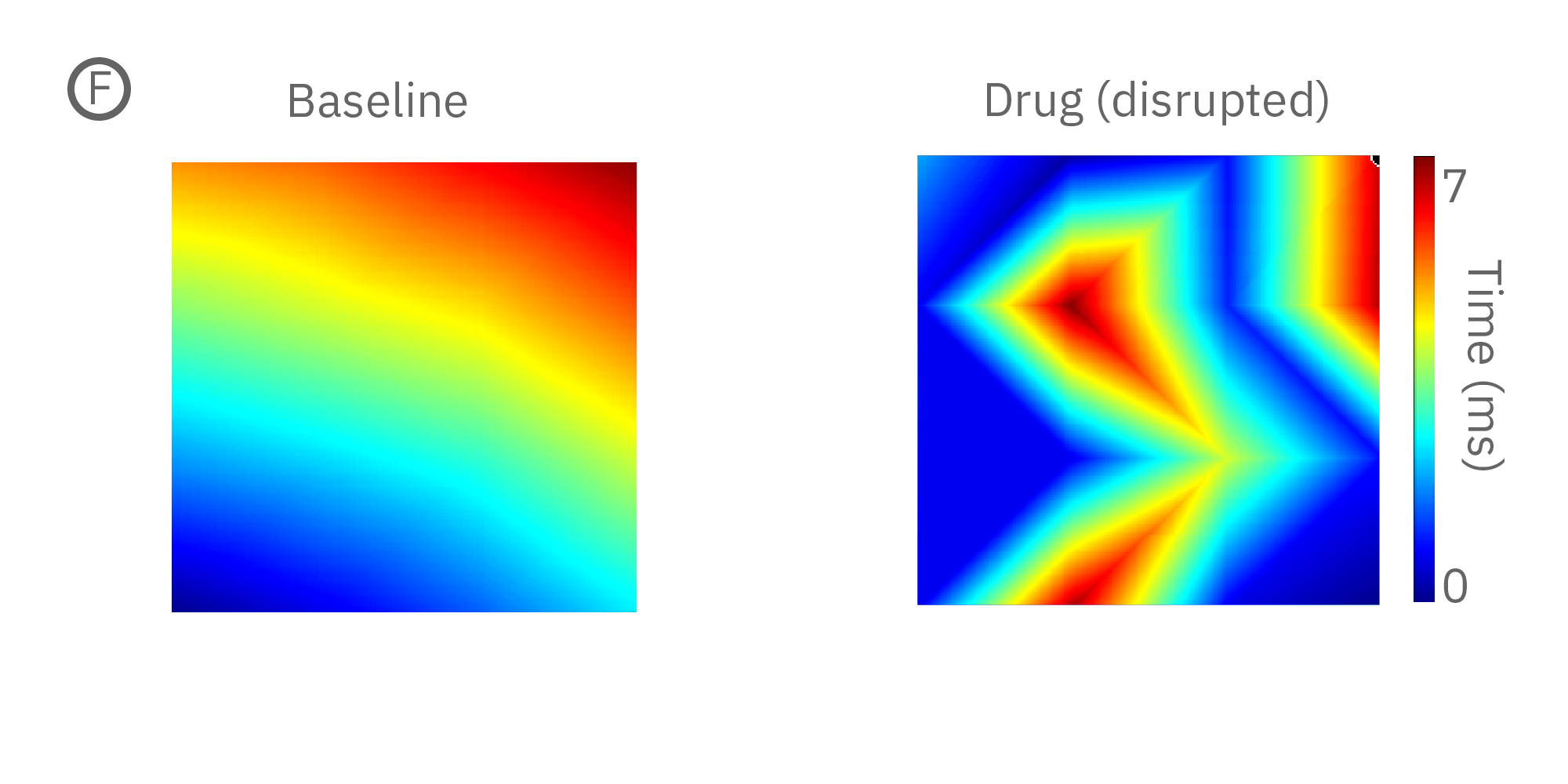
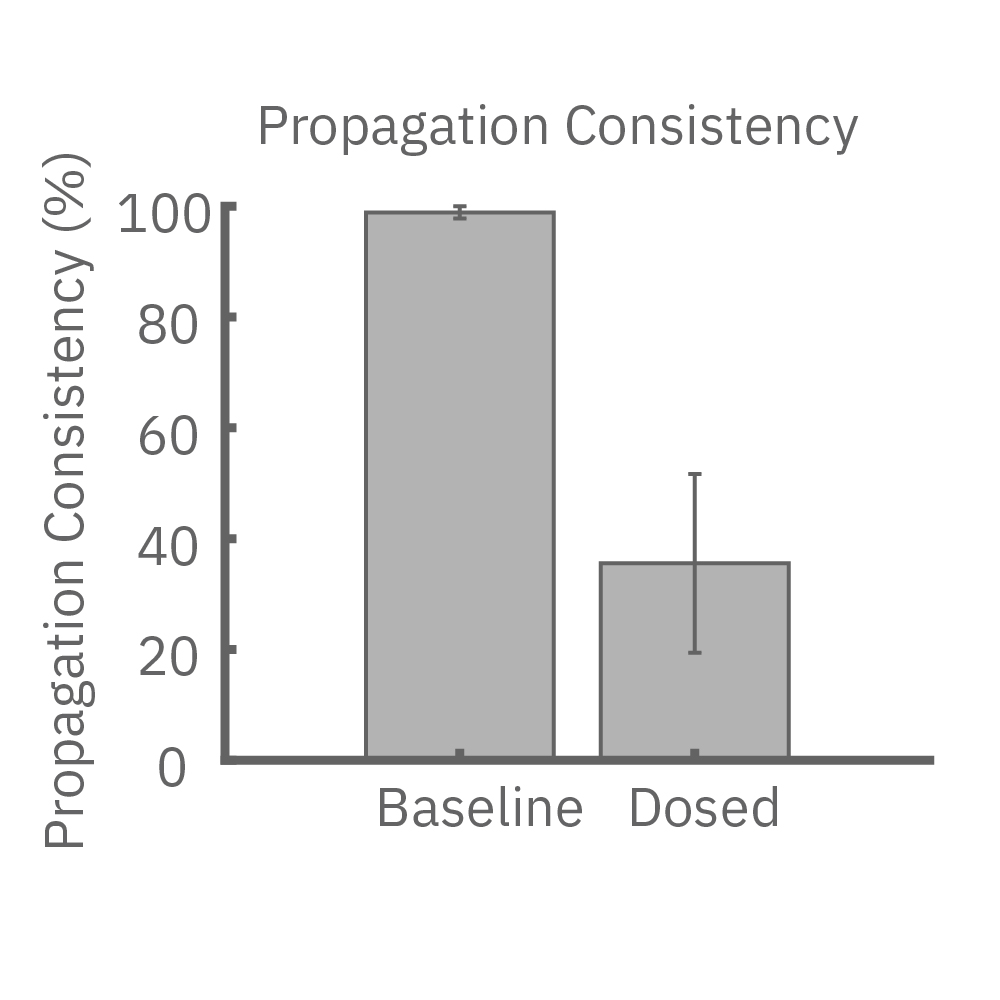
(E) iPS細胞由来心筋細胞に、Na+チャネルブロッカーを投与した際の伝播マップ(左>中央)と伝播速度の変化 (右)。濃度依存的な伝搬速度の減少が見られた。
(F) 薬剤による伝播パターンの不規則化の例(左>中央)。右図は薬剤投与によるビート毎の伝搬規則性の減少を示す。
同一電極を用いた構造的・機能的心毒性の検証
心毒性は心臓の機能のみならずその構造にも影響を及ぼします。Maestro Pro/Edgeでは、MEA測定による心筋細胞の電気的な活動の検出に加え、同電極を用いて細胞の生存率(電極への被覆によるインピーダンス変化)を測定することが可能です。同一サンプルからの両測定により多くの情報が得られます。また、非侵襲電極による測定は、数日間に渡る慢性評価にも最適です。
Learn more about MEA Viability.
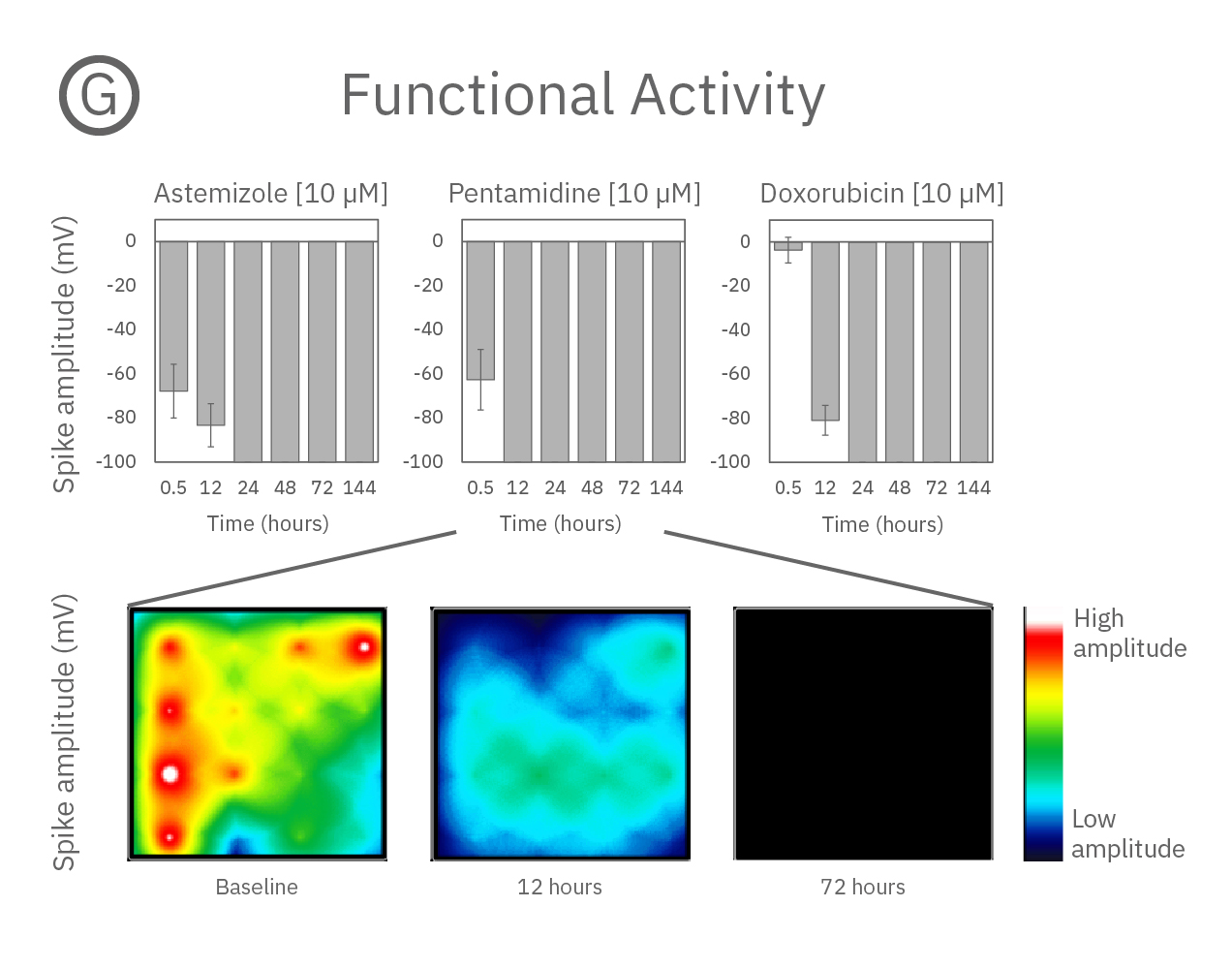
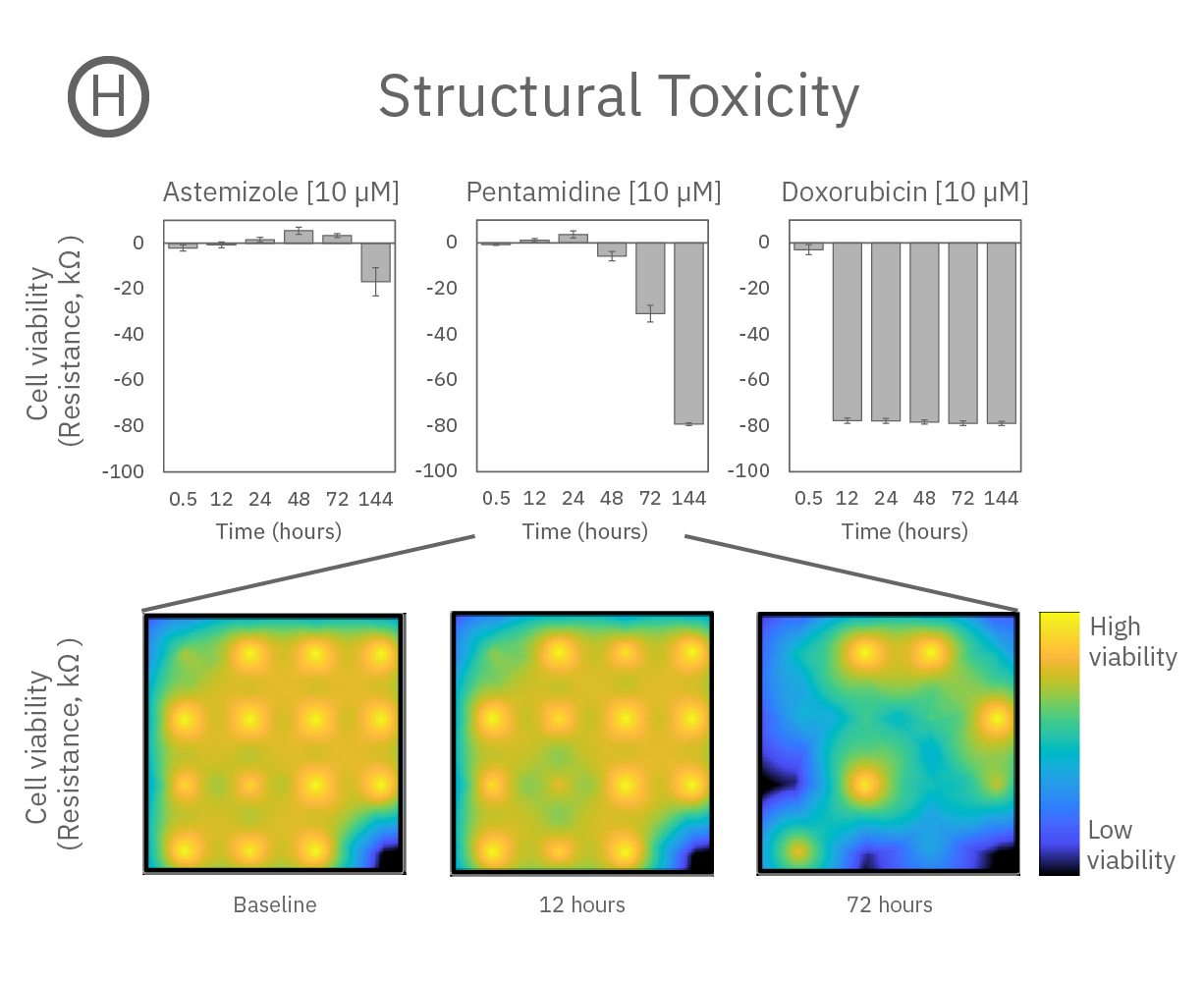
心機能に影響を及ぼす3種の薬剤 (Astemizole、Pentamidine、Doxorubicin) 投与による経時的な変化を検証した。
(G) 細胞外電位測定によるスパイク振幅値の経時的変化を示す。3種類の薬剤にて、時間の経過と共に振幅値は減少及び消失し、強い心毒性が示唆された。
(H) Viability (インピーダンス)の経時的変化を示す。強い毒性は、Pentamidine (hERG trafficking 阻害剤) とDoxorubicin(抗がん剤)のみに見られた。また、細胞死に至る速さは薬剤毎に異なり、Doxorubicinでは24時間以内であった一方、pentamidineでは6日間を要した。
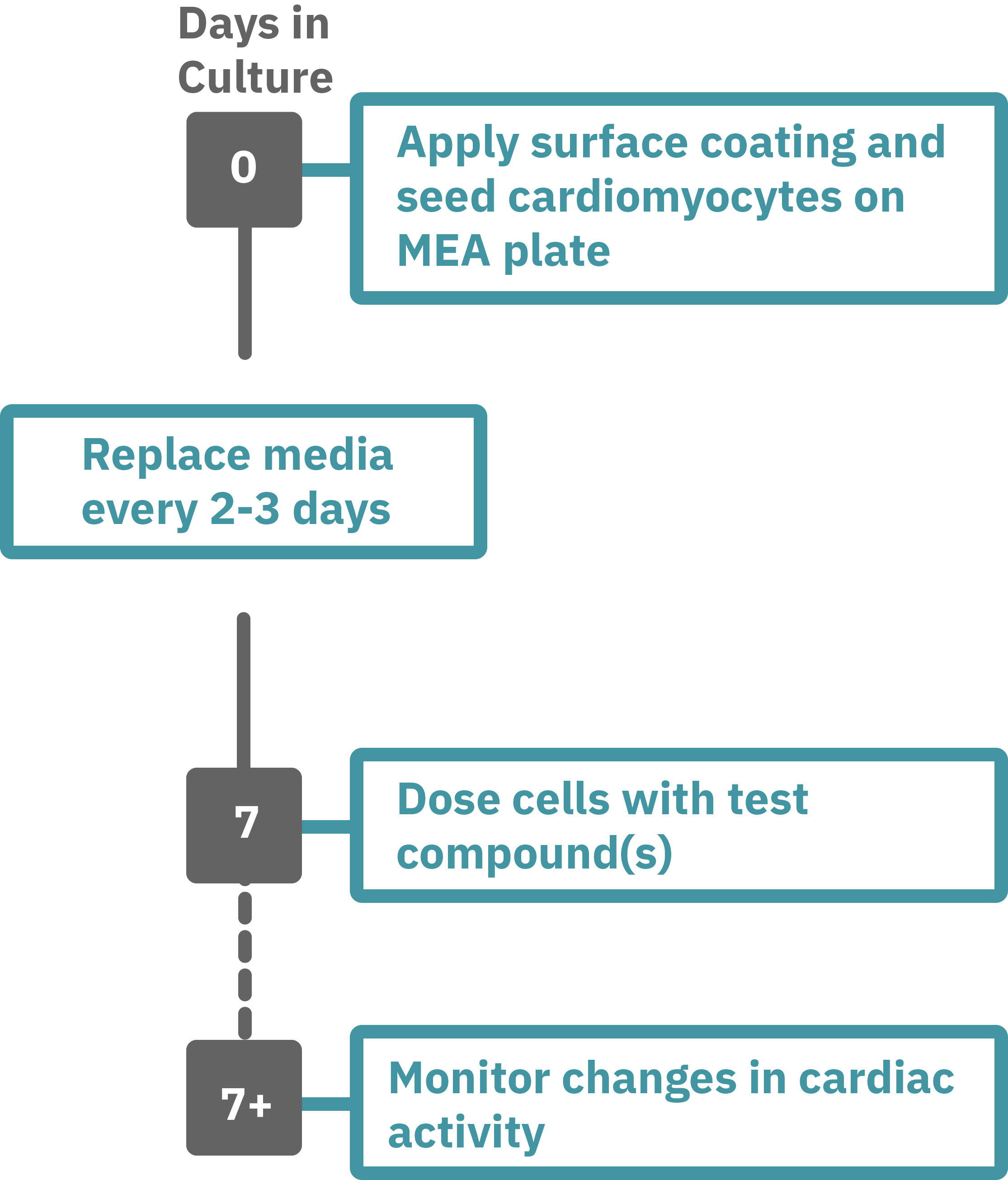
Maestro Pro/Edge による心毒性評価は非常に簡単です。
コーティングされたMEAプレート上に直接心筋細胞を播種します(Day 0)。2-3日毎に培地交換をしながら、一定期間細胞を培養します。
データ測定日 (例: Day 7+) にプレートを Maestro に搭載し測定を行います。データは付属のソフトで解析可能です。
Maestro MEA による心毒性・安全性評価:特徴
-
1システムで4種類のアッセイ - Maestro Pro/Edgeでは、1枚のプレートで次の4種類のアッセイが行えます。[1] 細胞外電位応答 [2] シグナル伝播 [3] Contractility (弛緩収縮評価) [4] LEAP (活動電位形態シグナル)
-
ラベルフリー・リアルタイムで細胞の電気的な活動を測定 - プレート上の電極を用いて心筋細胞の活動電位を測定します。ラベルフリー、リアルタイムな測定は、試薬など2次的要因によるゆがみがなく、細胞の変化をより正確にとらえます。また、数日から数週間に渡る慢性評価にも最適です。
-
同一プレート上で培養から測定まで - MEAプレート上で直接細胞を培養し、測定します。環境要因による変化を最小限にとどめながら、細胞への負担が少ない状態で、細胞の変化を検出することができます。
-
安定した環境下での実験 – 温度・CO₂ 濃度は、装置搭載のコントローラで自動制御されます。また、Maestro は外来ノイズ・振動に影響されにくい設計になっています。安定した環境で毎日安心して実験に望めます。
-
簡単操作 - 電気生理未経験の方でも簡単に実験が行えます。MEAプレート上に細胞を培養し、装置に搭載するだけで、心筋細胞の電気的な活動の測定が可能です。付属のソフトウエアパッケージを用いて、数クリックで複数の指標に基づいた解析結果が得られ、結果の作表まで行うことができます。
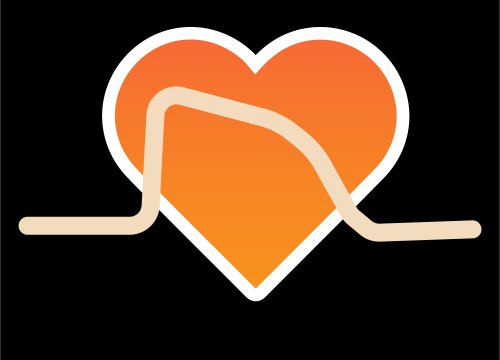
Cardiac LEAP
Show Full DetailsLEAP: For cardiac action potential recordings
The cardiac action potential is an electrical signal characterized by the depolarization across the cell membrane of cardiomyocytes, resulting in contraction of the heart. The shapes of an action potential provide vital information about the cardiomyocyte biology, health, and response to a drug. However, measuring the cardiac action potential traditionally requires invasive techniques, such as manual patch clamp, or labels, such as voltage-sensitive dyes.
LEAP technology enables non-invasive, label-free monitoring of the cardiac action potential in a high-throughput real-time format. LEAP can be used for quantification of action potential morphology, repolarization irregularities such early after depolarizations (EADs), and arrhythmic risk factors such as triangulation. Key metrics, such as rise time, action potential duration (APD), triangulation ratio, and percentage of beats with EADs are all automatically detected by LEAP.
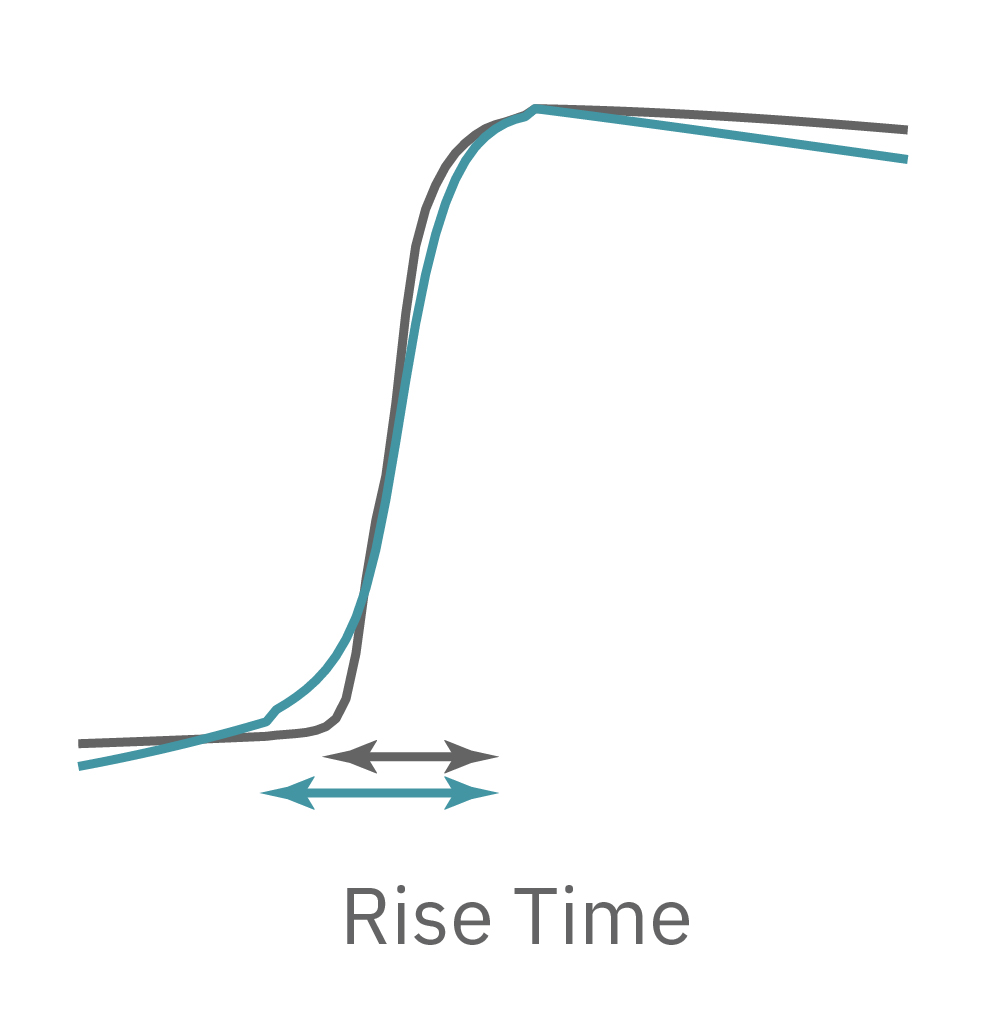
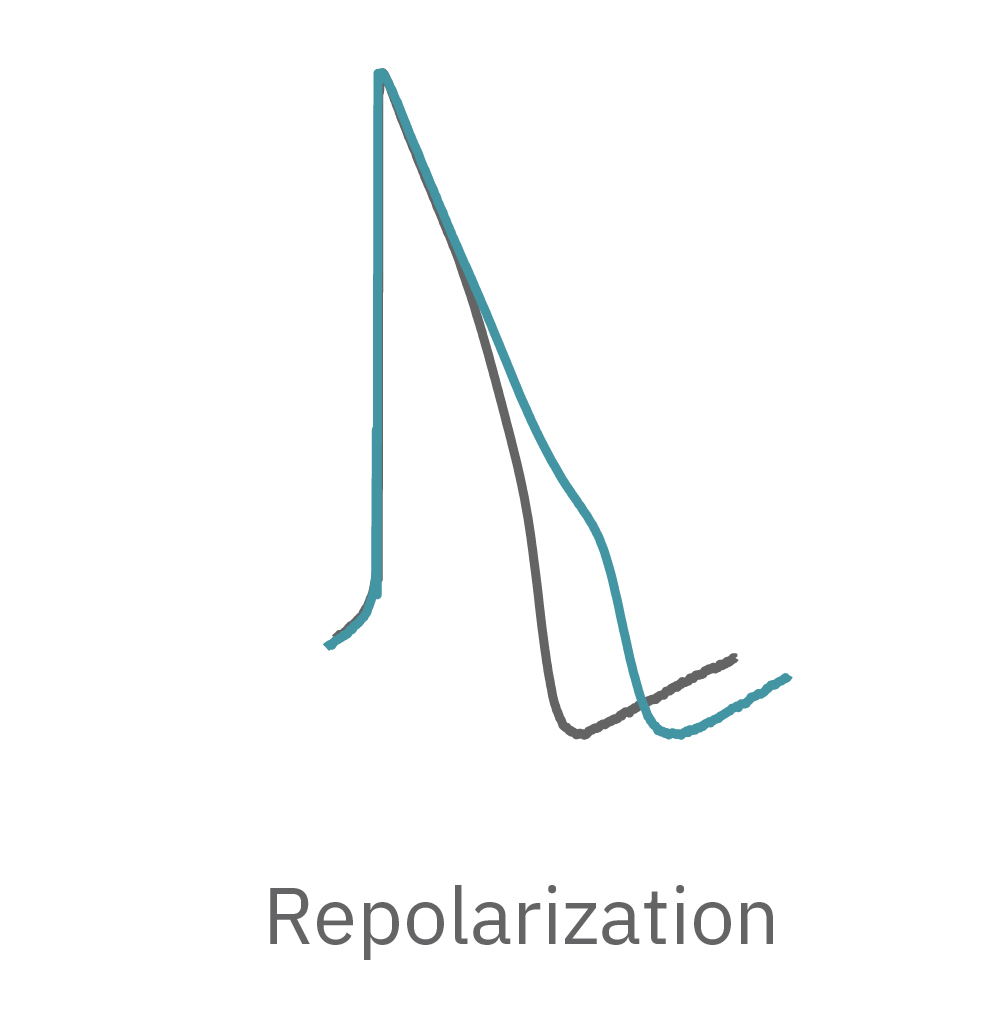
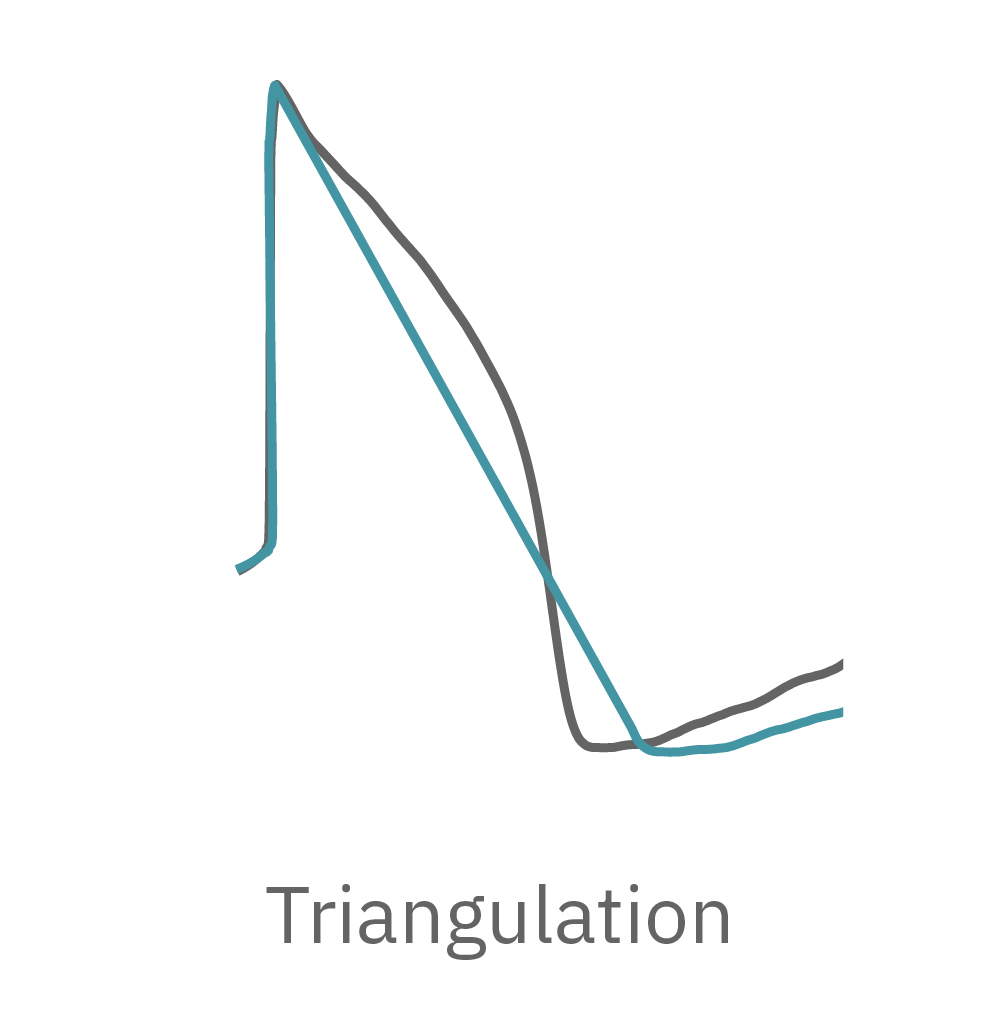
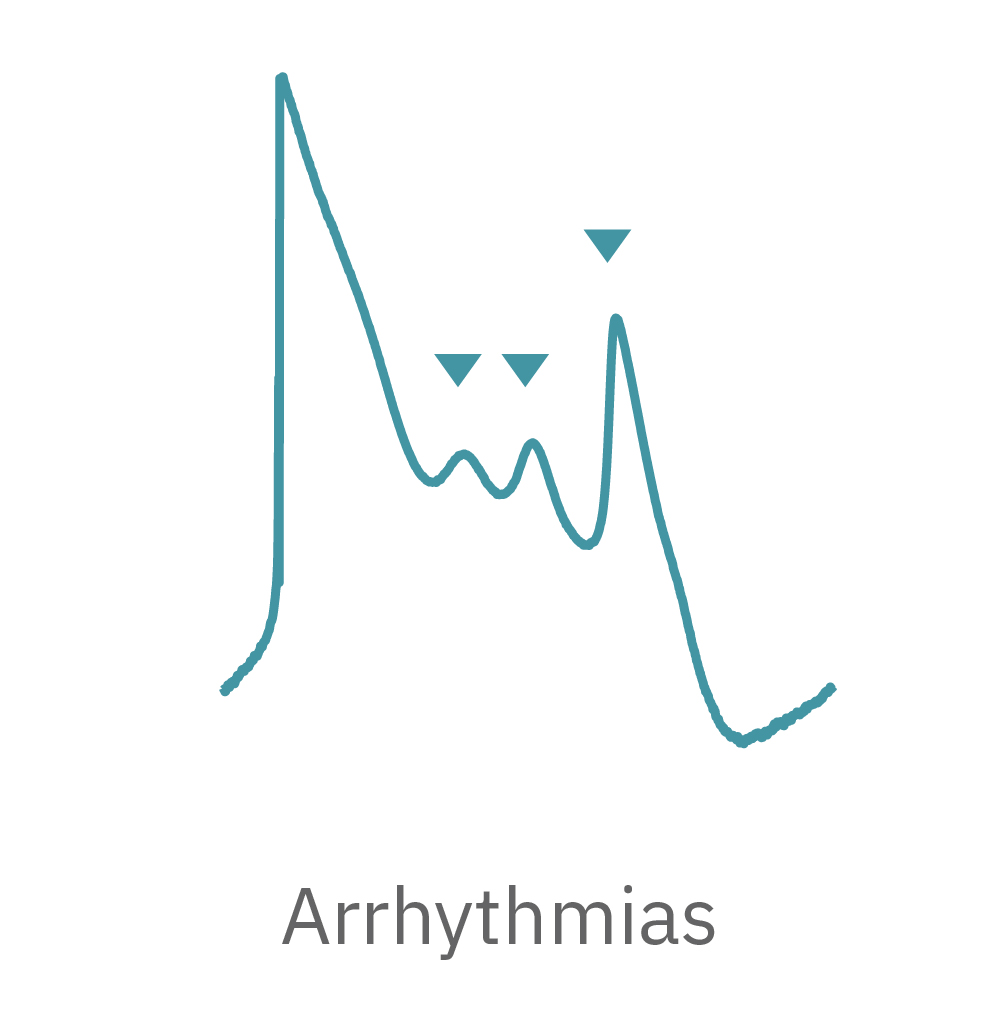
How does LEAP work?
LEAP stands for local extracellular action potential. The theory behind LEAP is similar to that of patch clamp, where the recorded signal amplitude is proportional to the sealing resistance between the electrode and the cell.
In contrast to a field potential signal, LEAP induction increases the coupling between the cells and electrode, enabling the measurement of a much larger action potential signal. The increased cell-electrode coupling is stable for 10-20 minutes or longer, allowing extracellular monitoring of the cardiac action potential without disrupting the underlying biology with dyes or invasive electrodes.
"The LEAP assay addresses an important gap in the field, namely providing a non-invasive solution in recording high quality action potentials from cardiac cells using a high throughput format. The LEAP assay may be a game changer."
Bernard Fermini, PH.D.
Chief Scientific Officer, Coyne Scientific
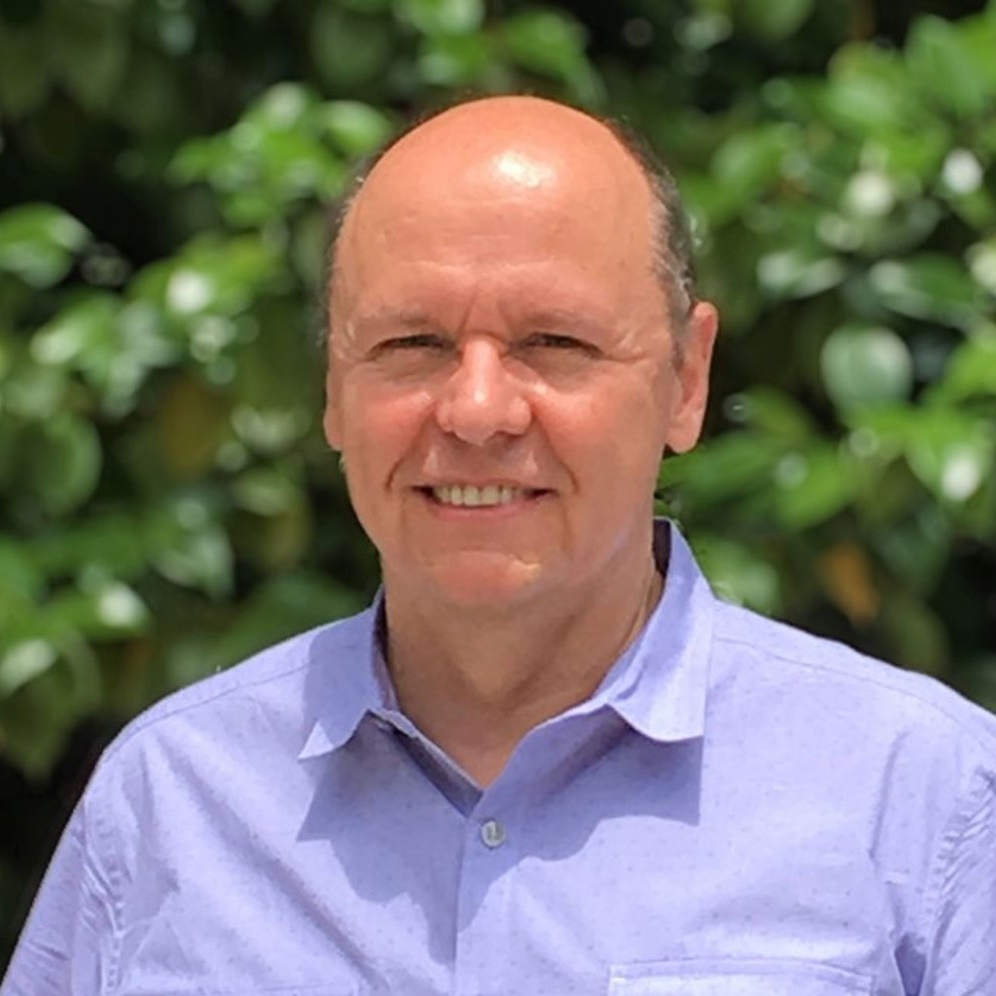
How does LEAP differ from the classic field potential?
The field potential has long been the standard for high-throughput in vitro cardiac electrophysiology. The field potential derives from the underlying cardiac action potential, but more closely resembles the low amplitude clinical electrocardiogram (ECG) signal. The initial depolarization phase is seen as a sharp spike, similar to the QRS complex, followed by the slow repolarization analogous to the T wave. Although the field potential has proven highly effective for high-throughput drug screening and other applications, the field potential shape can obscure complex repolarization irregularities and limit comparisons to gold standard manual patch clamp recordings.
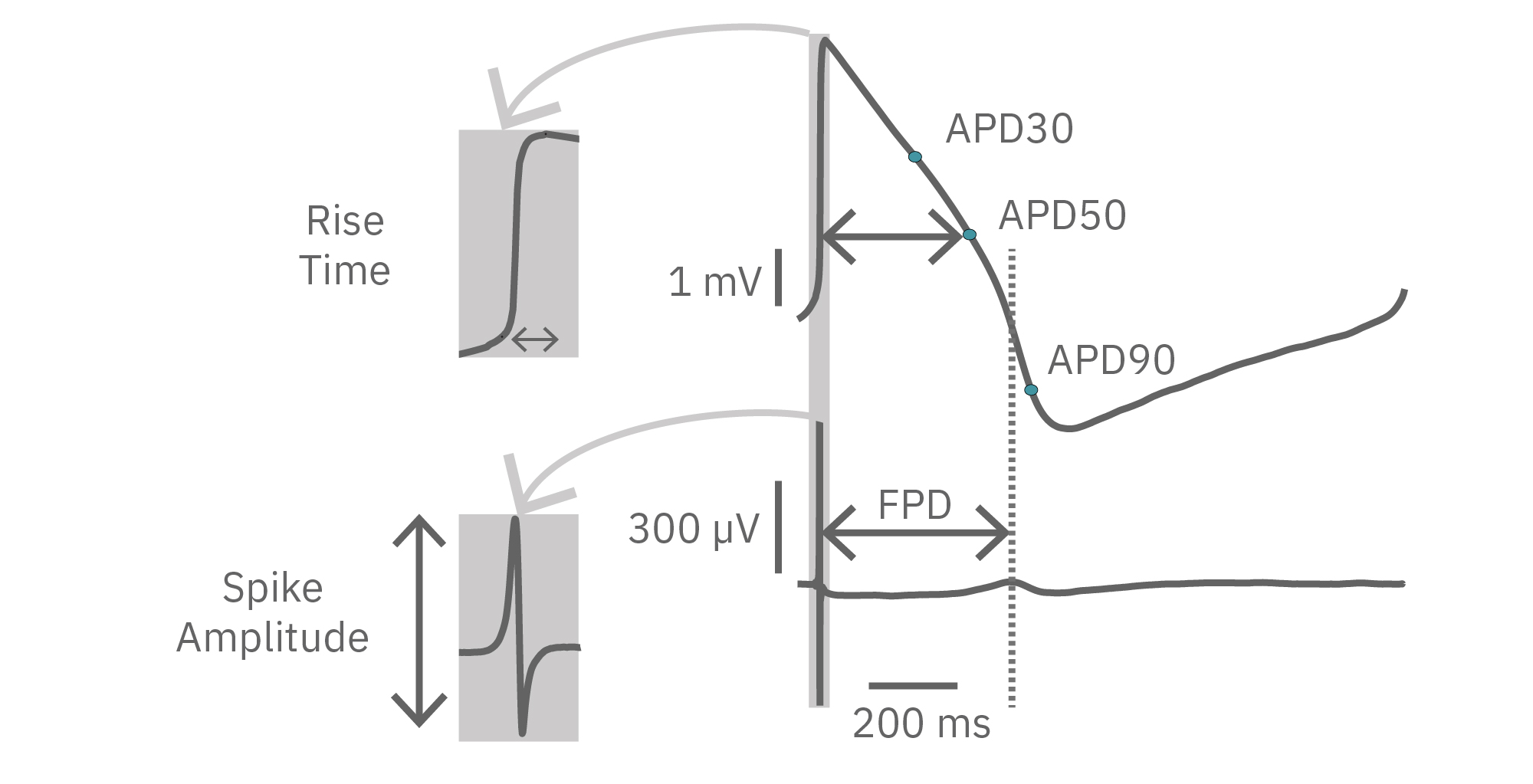
The LEAP signal accurately reflects the shape and duration of the underlying action potential. The large signal allows for automated detection and classification of arrhythmic events, such as notched EADs, rolling EADs, or ectopic beats. LEAP also provides metrics not available from the field potential, such as rise time and triangulation.
Because LEAP operates on each electrode independently, field potential and LEAP signals can be recorded from the same well simultaneously, providing a direct mapping between features of the field potential and the action potential. In this way, LEAP enables confirmation and automation of feature detection.
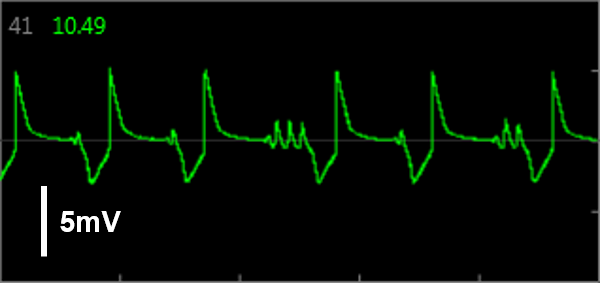
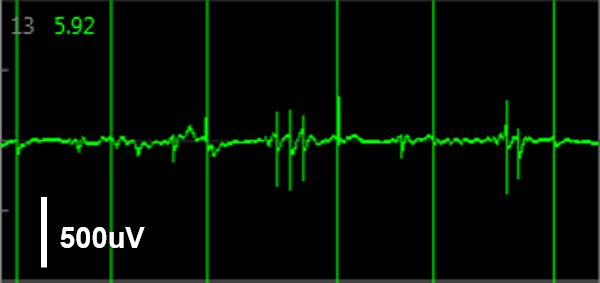
Simultaneous LEAP and field potential (FP) measurements establish translation between FP and LEAP signals. LEAP was induced on half of the microelectrodes in each well of a MEA 48-well plate of iCell CM2. Example LEAP and FP signals from the same well when dosed with E-4031. Depolarization aligned between the LEAP and FP signals, but EADs could be more reliably detected in the LEAP trace.
Advantages of LEAP
Due to the larger features of the signal, the LEAP assay is robust against pharmacological manipulations that can render field potential features difficult to detect.
For example, sodium channel blockers cause a detectable change in spike amplitude. At higher doses though, the spike amplitude can become too small to detect. However, rise time prolongation in the LEAP signal can still be easily measured even at high doses. Similar effects can be seen at high doses of hERG blockers when hERG block begins to impact the resting membrane potential.
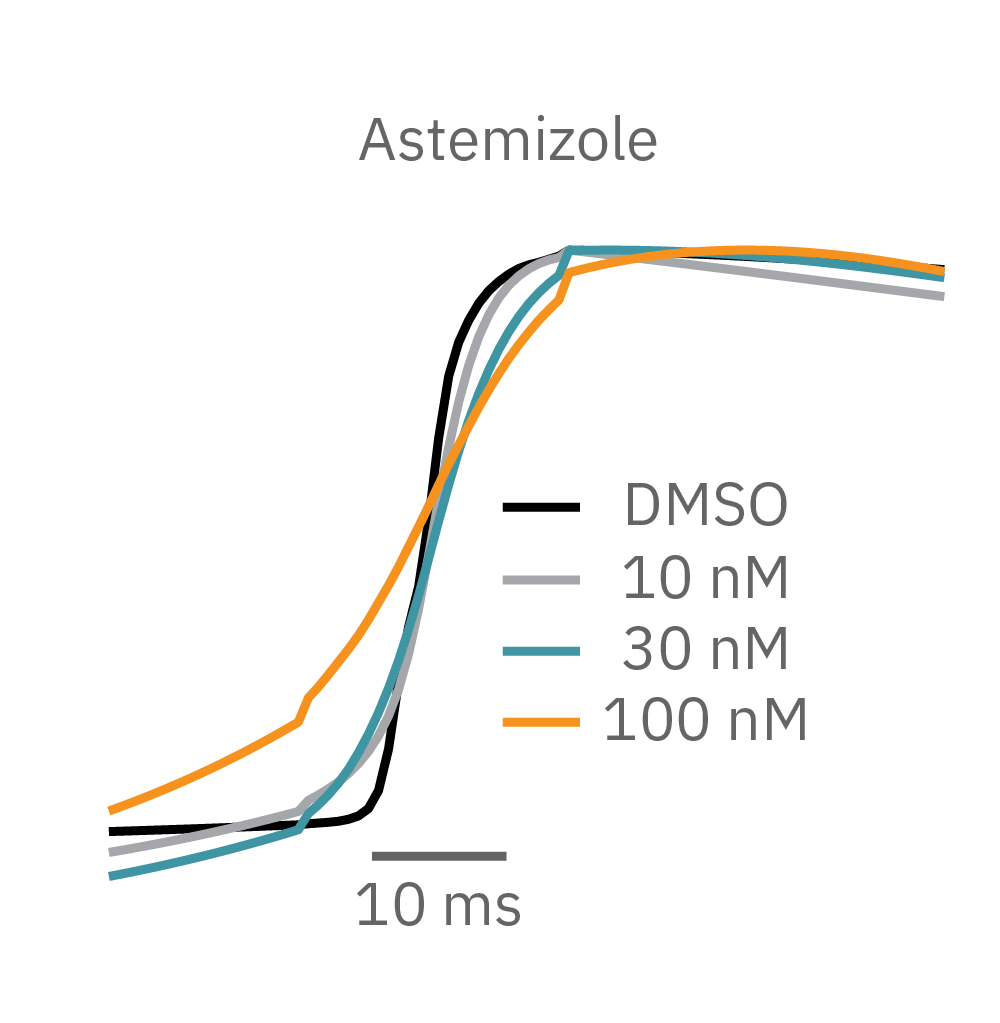
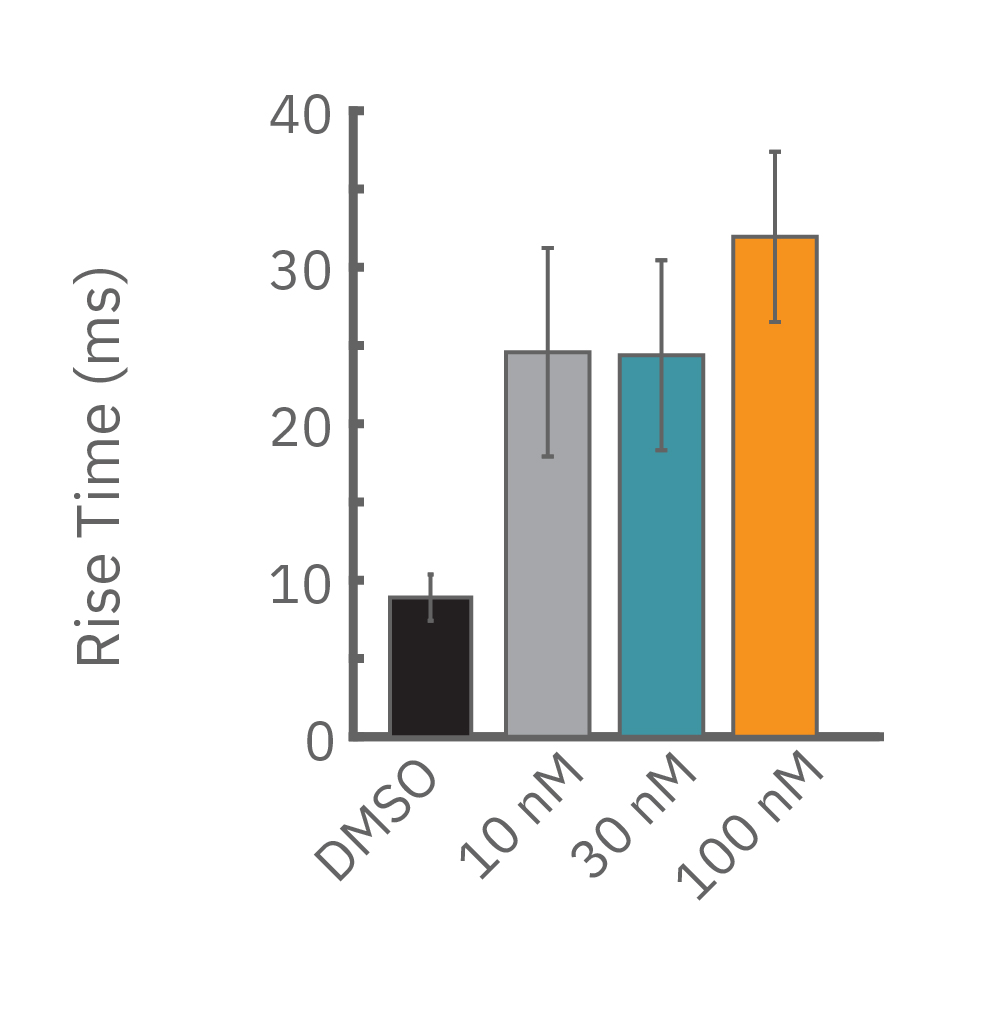
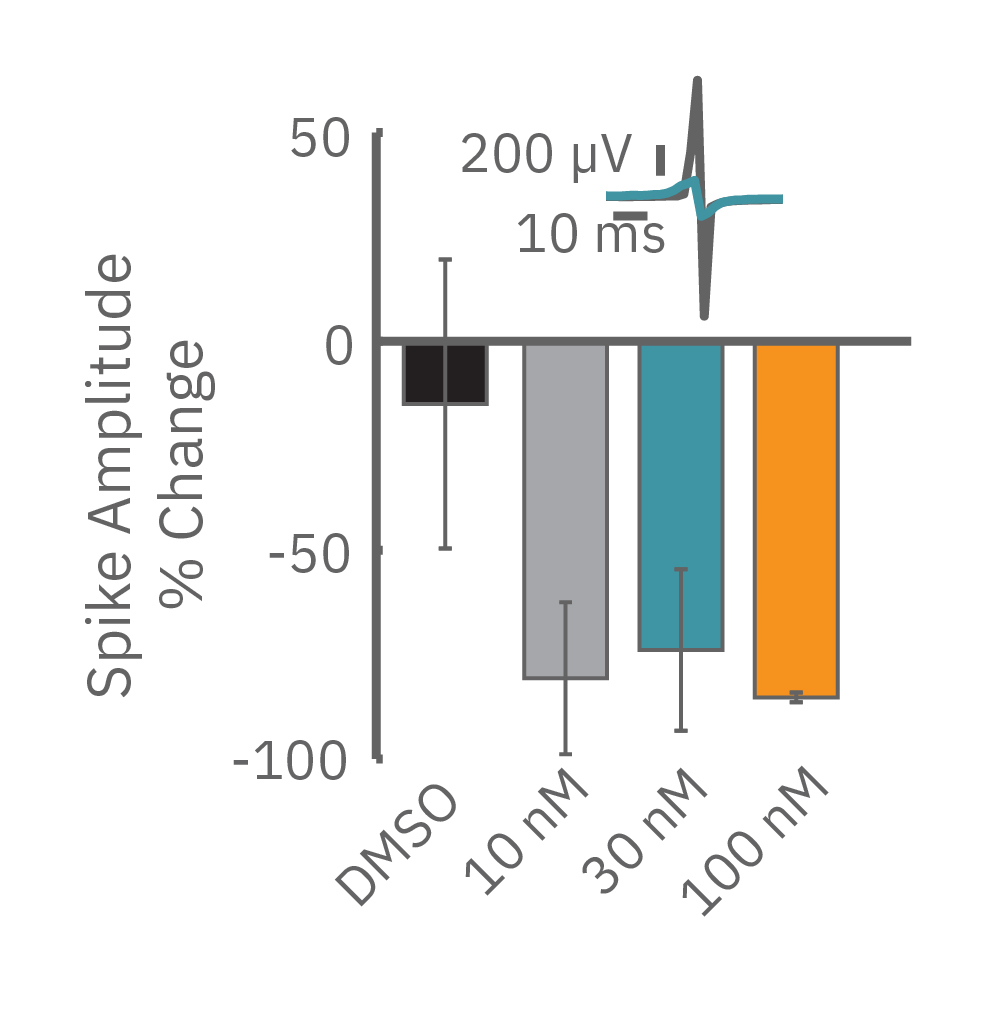
Similarly, compounds, such as terodiline, that induce triangulation can flatten the field potential repolarization feature, referred to as the “T wave”. The resulting broad, small amplitude T wave is difficult to detect and quantify. In contrast, triangulation is readily detectable and quantifiable in the LEAP signal.
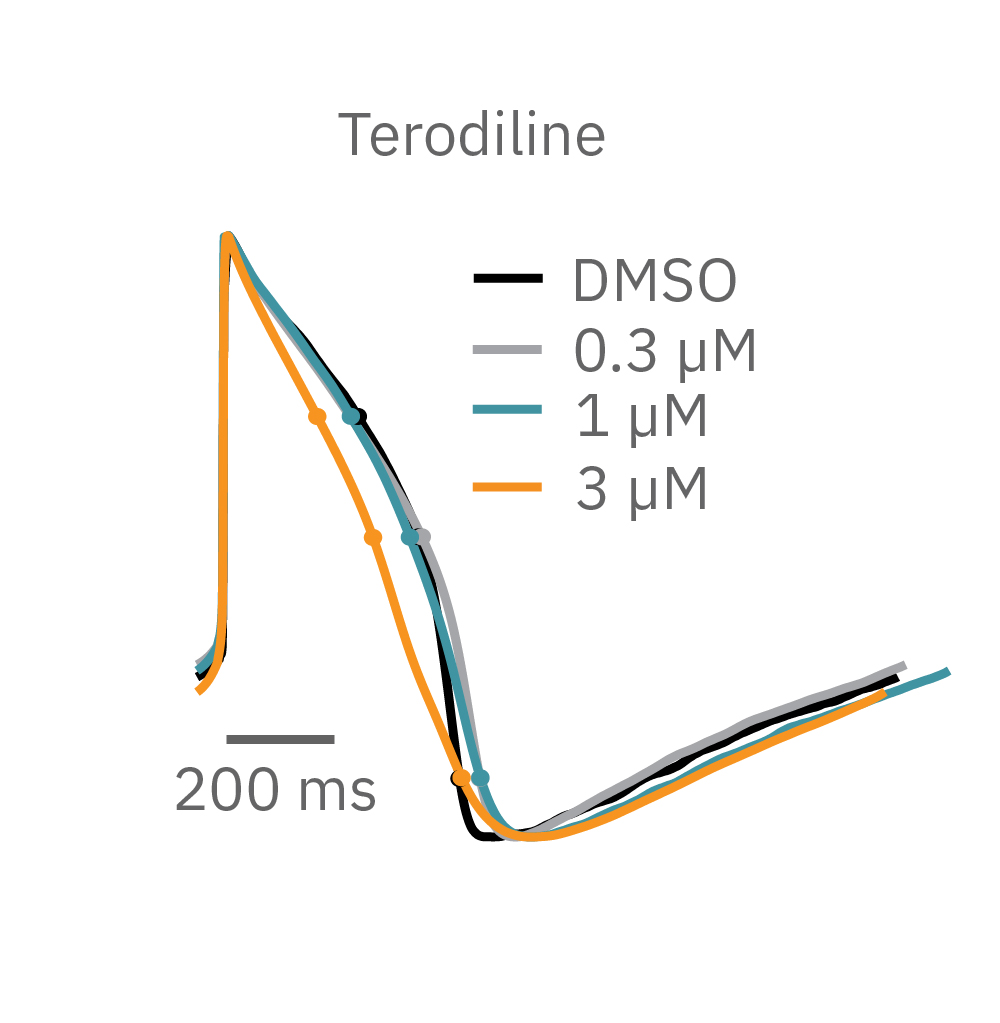
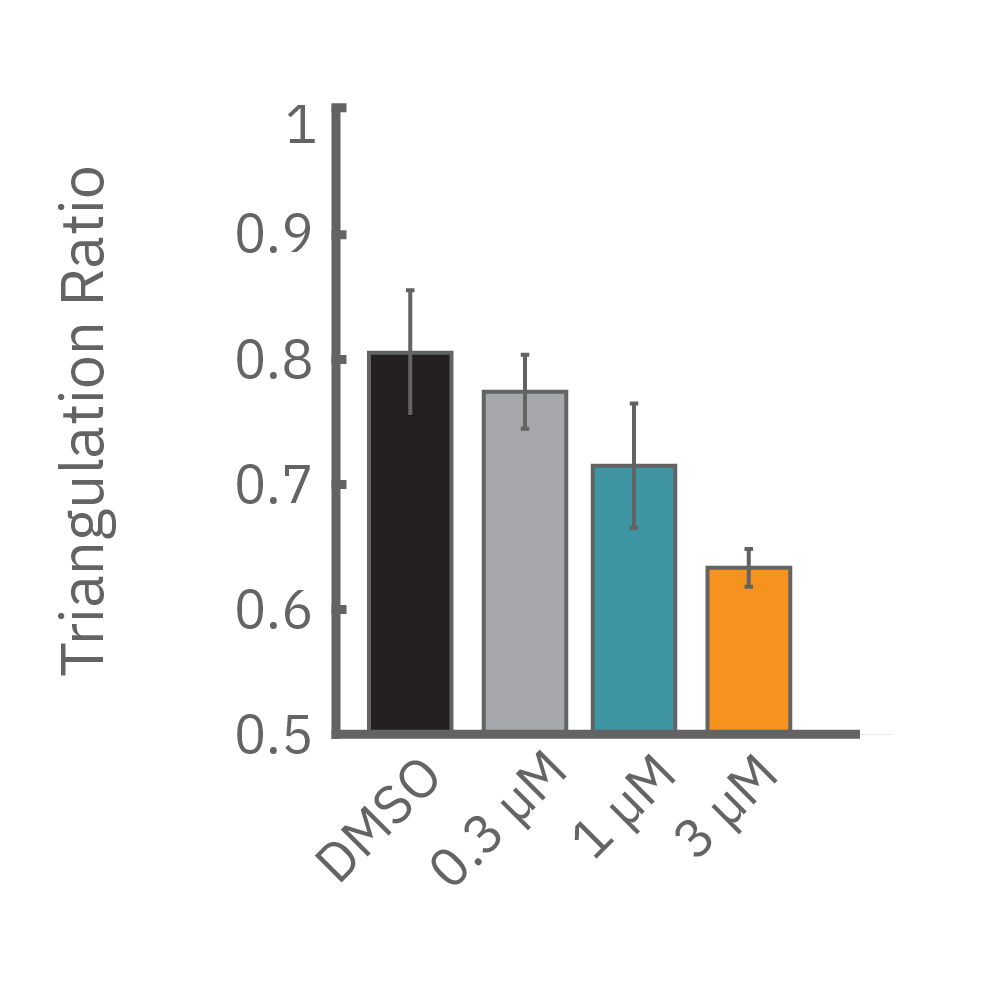
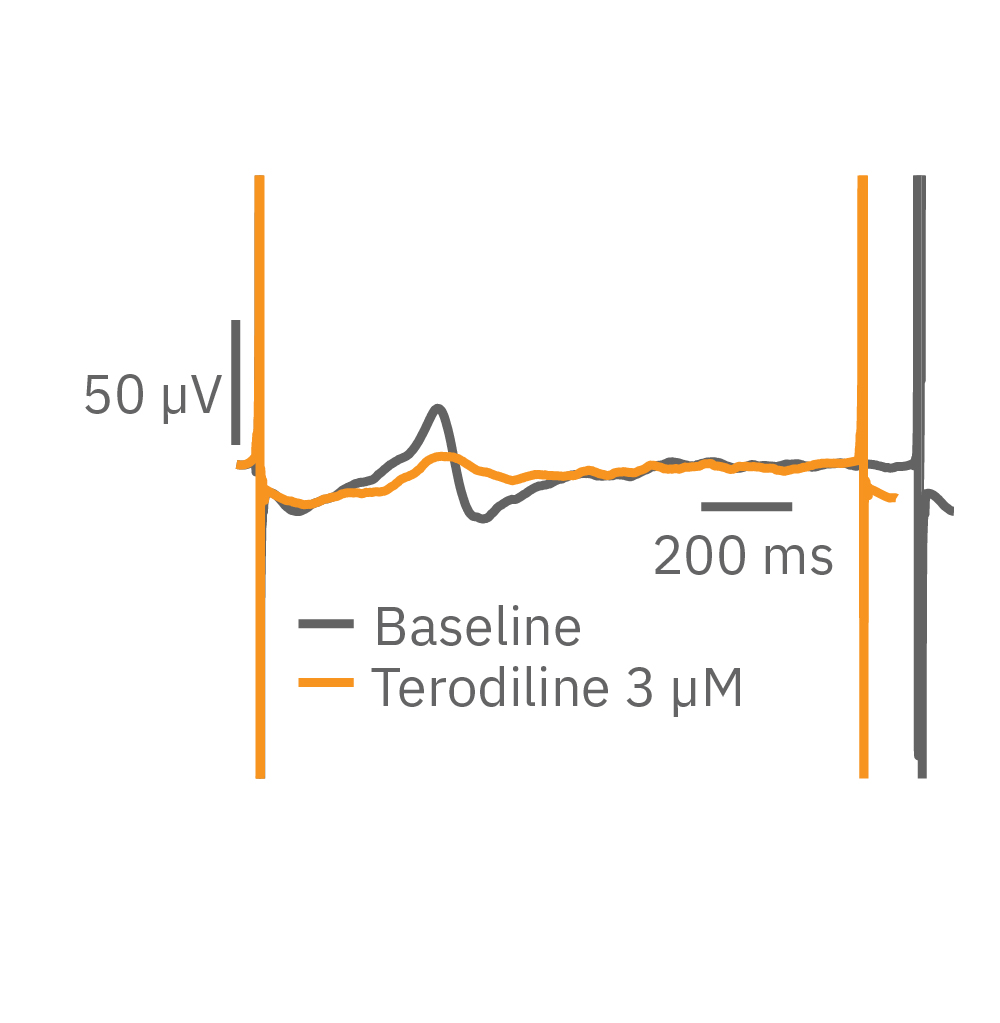
LEAP applications
LEAP is ideal for capturing even small differences in action potential morphology in cardiac health and disease and in response to compounds. LEAP is a powerful tool for many applications including:
- Automated APD and EAD detection for high throughput drug screening
- Predicting arrhythmic risk for cardiac safety and cardiotoxicity testing
- Characterization of action potential morphology in human induced pluripotent stem cell-derived (hiPSC) cardiomycoytes
- Studying the effects of genetic manipulation on cardiac electrophysiology
- Comparing cardiac biology in healthy and diseased states
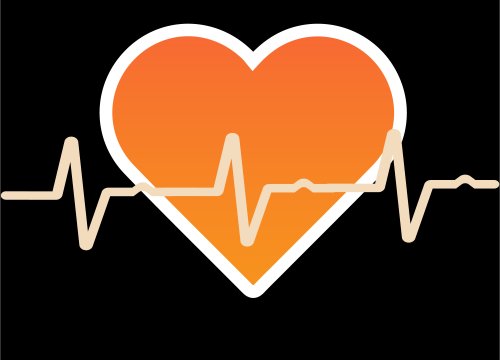
Cardiac MEA
Show Full DetailsWhat is a microelectrode array (MEA)?
Microelectrode arrays (MEA), also known as multielectrode arrays, contain a grid of tightly spaced electrodes embedded in the culture surface of the well. Electrically active cells, such as cardiomyocytes, are cultured on top of the electrodes. When neurons fire action potentials, the electrodes measure the extracellular voltage on a microsecond timescale. As the cells attach and connect with one another, an MEA can simultaneously sample from many locations across the culture to detect propagation and synchronization of cardiac activity across the syncytium.
That’s it, an electrode and your cells. No dyes, no incubation steps, no perfusion, no positioning things just-so; just your cells in a well. Because the electrodes are extracellular (they do not poke into the cells), the recording is noninvasive and does not alter the behavior of the cells, you can measure the activity of your culture for seconds or even months!
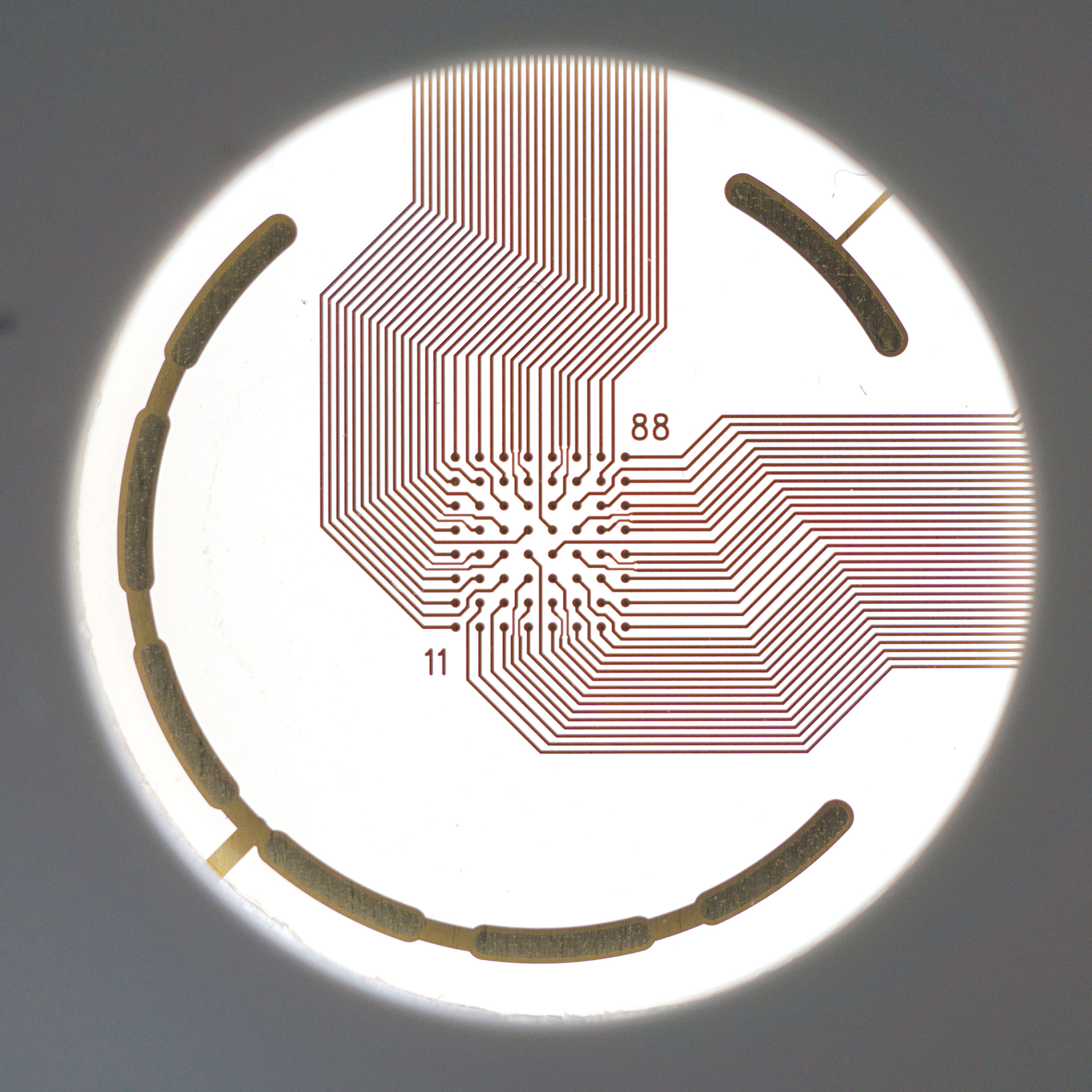
An MEA of 64 electrodes embedded in the substate at the bottom of a well.
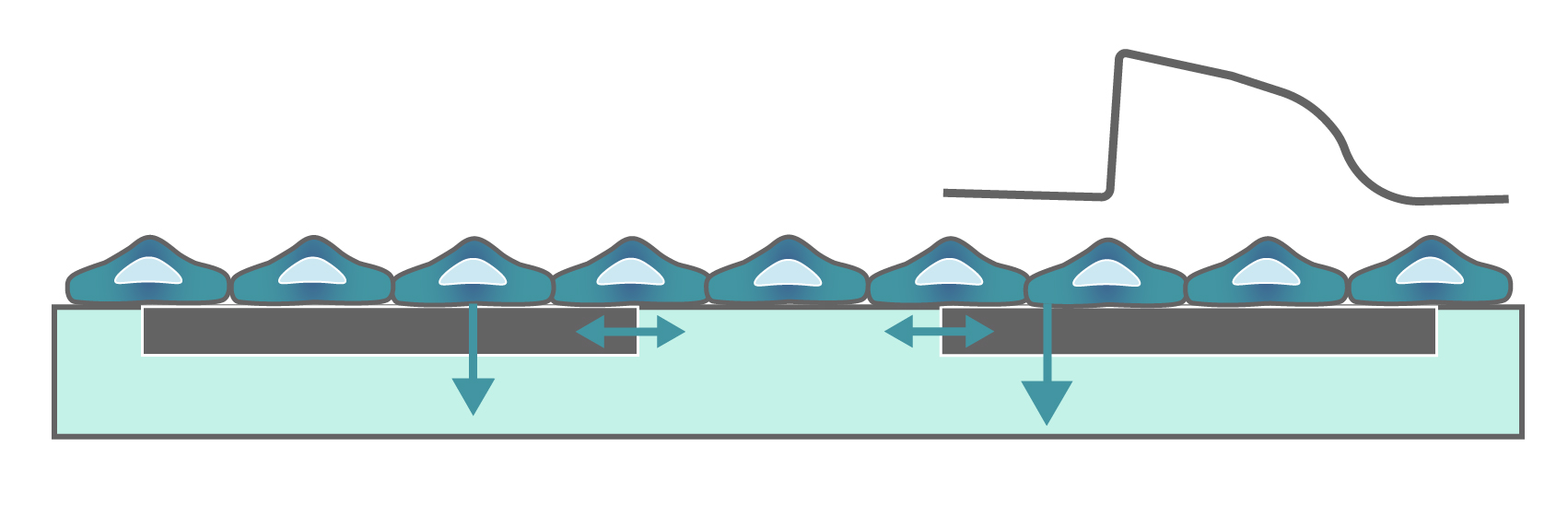
Cardiomyocytes attach to the array and form a network. The microelectrodes detect the action potentials fired as well as their propagation across the network.
Heartbeats in a dish
When cardiomyocytes are cultured on top of an MEA, they attach and connect to form a spontaneously beating sheet of cells, called a syncytium. When one cardiomyocyte fires an action potential, the electrical activity propagates across the syncytium causing each cell to fire and then contract. The electrodes detect each individual action potential and contraction, as well as the propagation of this activity across the array.
The propagating electrical signal is detected by the electrodes as an extracellular field potential. The field potential derives from the underlying cardiac action potential, but more closely resembles a clinical electrocardiogram (ECG) signal. The initial depolarization phase is seen as a sharp spike, similar to the QRS complex, and the slow repolarization is seen as a small slow spike, like a T-wave. The time from the depolarization to repolarization is termed the field potential duration (FPD) and is a key metric in predictive cardiotoxicity screening assays.
While most record the cardiac field potential, the Maestro Pro and Edge MEA systems can also measure local extracellular action potentials, or LEAP. LEAP induction increases the coupling between the microelectrodes and the cardiomyocytes, transforming the extracellular signal from a field potential to an action potential. LEAP provides additional and complementary metrics such as rise time, action potential duration (APD), triangulation, and automated early after depolarization (EAD) detection.
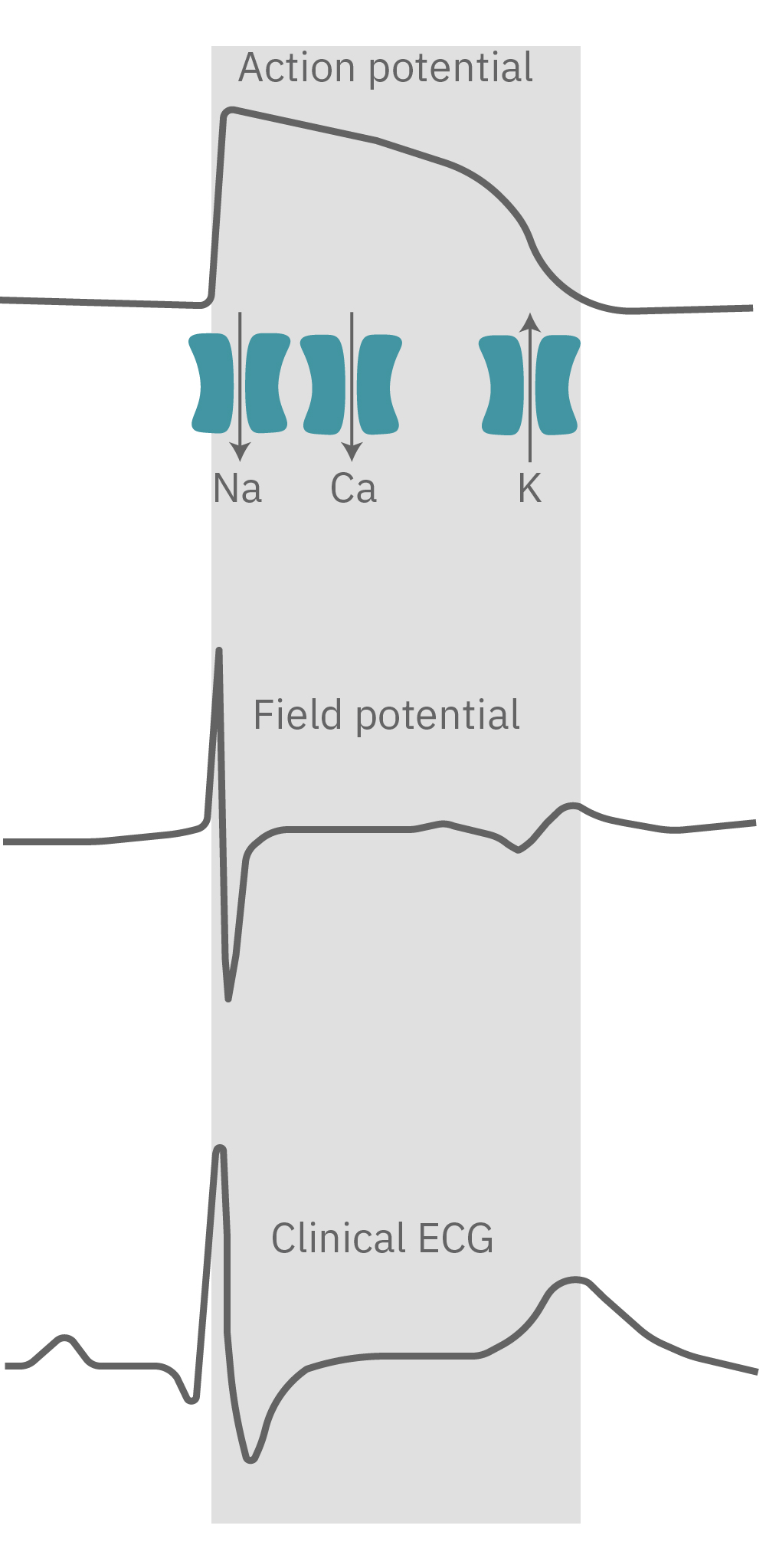
The cardiac action potential propagates from cell to cell across the syncytium. The MEA detects this activity as an extracellular field potential, which closely resembles the clinical ECG.
Do more with multiwell
Axion BioSystems offers multiwell plates at many throughputs, from 6-wells to 96-wells, with an MEA embedded in the bottom of each well. Each well represents its own unique cell culture and conditions, creating up to 96 experiments on one plate. Multiwell MEA allows you to study complex human biology in a dish, from a single cell firing to network activity, across many conditions and cell types at once.